the Creative Commons Attribution 4.0 License.
the Creative Commons Attribution 4.0 License.
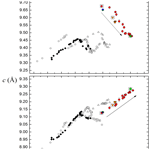
Evidence of the existence of the As4S6 molecule produced by light exposure of alacranite, As8S9
Paola Bonazzi
Laura Chelazzi
Matteo M. N. Franceschini
Giovanni O. Lepore
Marta Morana
Giovanni Pratesi
Alice Taddei
Matteo Zoppi
Silvio Menchetti
The As4S6 molecule, although theoretically predicted and supposed to occur in amorphous arsenic sulfides, was missing in the reported structures of crystalline As chalcogenides; the thermodynamic stable phase for this stoichiometry, in fact, is that of the mineral orpiment, which shows a layered structural arrangement based on trigonal AsS3 pyramids. Here we report the first possible occurrence of the As4S6 molecule together with the other known As4Sn (n=3, 4, 5) molecules randomly replacing each other in the crystalline structure of a new monoclinic product obtained by the light-induced alteration of the mineral alacranite, As8S9. Our findings are based on single-crystal X-ray diffraction experiments. The fact that As4S6 formed in a crystalline light-induced alteration product could indicate a heretofore unknown role of this molecule in the photoinduced changes of the physico-chemical properties of both bulk glasses and thin films in the As–S system, widely studied in optics and optoelectronics.
- Article
(2528 KB) - Full-text XML
- BibTeX
- EndNote
In the As–S system (Emelina et al., 2007), several compounds with different As:S ratios are known as minerals. They include duranusite, As4S; α- and β-dimorphite, As4S3; realgar and pararealgar, As4S4; uzonite, As4S5; alacranite, As8S9; and orpiment, As2S3 (Bonazzi and Bindi, 2008). The high-temperature polymorph β-As4S4, i.e. bonazziite, was also found as a natural phase (Bindi et al., 2015). Non-stoichiometric sulfides with chemical composition ranging from As4S4 to As8S9 have also been reported (Bonazzi et al., 2003a). Most of the arsenic sulfides have a crystal structure consisting of a packing of cage-like, covalently bonded As4Sn (n=3, 4 and 5) molecules held together by weak interactions of van der Waals characters (Bonazzi and Bindi, 2008). In contrast, duranusite (Bonazzi et al., 2016), with As:S , and orpiment (Mullen and Nowacki, 1972), which fits the atomic ratio As:S , exhibit a layered structural arrangement, despite the fact that ab initio quantum chemical calculations (Kyono, 2013) predicted the presence of molecular groups for both minerals.
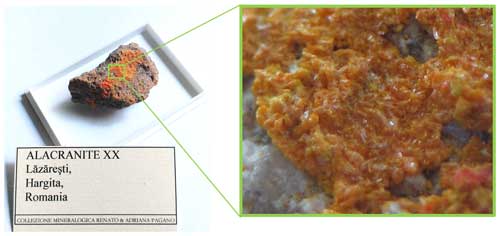
Figure 1Alacranite sample from the As-sulfide-bearing sandstones of Lăzăreşti, in the northern part of the Ciomadu volcanic area, Romania (sample size cm).
All these As sulfides, as well as bulk glasses and thin films in the As–S system, are of interest for their potential or actual application in optics and optoelectronics, due to reversible and/or irreversible photoinduced changes of their physico-chemical properties (e.g. Holomb et al., 2005; Němec et al., 2005). Furthermore, as arsenic sulfide minerals were largely used as pigments, the study of their light-induced alteration finds application in the examination and conservation of works of art (e.g. Trentelman et al., 1996; Vermeulen et al., 2015).
In particular, realgar (α-As4S4) and bonazziite (β-As4S4), the non-stoichiometric β-As4S4+x (Bonazzi et al., 2003a) and some adduct compounds (HgI2⋅ As4S4, Bonazzi et al., 2011; (HgBr2)3(As4S4)2, Zoppi et al., 2013) containing the realgar-type (r-type) molecules, undergo structural modifications when exposed to filtered light. During this process, the r-type As4S4 molecule irreversibly transforms into the pararealgar (p-type) As4S4 molecule (e.g. Bonazzi et al., 2006). Surprisingly, stoichiometric alacranite, which also contains r-type As4S4 molecules, does not experience any change when exposed to filtered visible light (Bonazzi et al., 2003b).
According to several authors (Bindi et al., 2003; Kyono et al., 2005; Naumov et al., 2007, 2010), such solid-state transformation occurs through a self-sustainable mechanism based on initial aerobic photo-oxidation (process 1: 5As4S4 (r-type) + 3O2→ 4As4S5 + 2As2O3) followed by release of an S atom by breaking an As–S–As linkage to form pararealgar: As4S5→ As4S4 (p-type) + S (process 2). Propagation of process 2 cyclically occurs by re-attachment of the free S to another r-type As4S4 molecule to form As4S5 and subsequent conversion to p-type As4S4+ S. It is not clear what determines the number of As4S5 molecules that can be stored within the structure and which are the main factors that may affect the kinetics of the autocatalytically induced conversion, which, as Naumov et al. (2007) pointed out, does not require further exposition to visible light. It is a fact, however, that different compounds containing r-type As4S4 molecules show different routes of transformation. A common feature for all of them is an expansion of the unit cell, which was ascribed to the increment of As4S5 molecules in light-exposed β-As4S4+x crystals (Bonazzi et al., 2006) and, to a much lesser extent, in realgar (Kyono et al., 2005; Naumov et al., 2010). On the other hand, the increase of the unit-cell volume was explained by a replacement of r-type with p-type molecules in the case of the HgI2⋅As4S4 adduct (Bonazzi et al., 2011). Similarly, it was demonstrated that, when the exposure to light takes place under anaerobic conditions, β-As4S4 (Zoppi and Pratesi, 2012) and realgar (Pratesi and Zoppi, 2015) transform to pararealgar without evidence of storing As4S5 molecules within the structure.
Conversely, the photoinduced alteration of the most S-rich among the studied non-stoichiometric β-As4S4+x crystal (x=0.35) resulted in a new phase with a Pccn crystal structure (Bindi and Bonazzi, 2007) consisting of discrete As4S5 molecules, with a very minor number – if any – of coexisting p-type molecules. Since the β-As4S4+x crystals had been supposed to consist of different proportions of β-As4S4 and As8S9 (i.e. alacranite) coherent crystalline domains (Bonazzi et al., 2003a), it was inferred that light exposure causes conversion to pararealgar or to the Pccn As4S5 structure starting from β-As4S4 or As8S9, respectively. This conclusion, however, was not in agreement with the lightening experiments previously carried out on alacranite from the type locality (Bonazzi et al., 2003b).
The recent finding of a new sample of alacranite gave us the opportunity to repeat the lightening experiments by varying the exposure conditions. The results are reported in this study.
A new sample (Fig. 1), belonging to the private collection of Renato and Adriana Pagano, coming from the As-sulfide-bearing sandstones of Lăzăreşti, in the northern part of the Ciomadu volcanic area, Romania, was found to be rich in alacranite, As8S9, having a diffraction quality higher than that previously described for that locality (Kristály et al., 2006). Several single crystals were checked by means of X-ray single-crystal diffraction: the unit-cell parameters were found to match roughly those of alacranite from the type locality (Bonazzi et al., 2003b), with unit-cell volumes ranging from ∼849 to ∼861 Å3. Noteworthy, the smaller the unit-cell volumes, the higher the diffraction quality was.
As smaller unit-cell volumes seemed symptomatic of a higher diffraction quality, a crystal (A1) suitable for the whole intensity data collection and light exposure experiments was selected on this basis (a=9.862(5), b=9.676(6), c=9.126(7) Å, β=102.84(5)°, V=849(9) Å3). The X-ray studies were performed with an Oxford Diffraction Xcalibur 3 diffractometer equipped with a Sapphire 2 CCD detector, with graphite-monochromatized MoKα radiation (λ=0.71073 Å), with working conditions 50 kV ×50 mA and with 100 s exposure time per frame; the detector-to-sample distance was 6 cm. The structure refinement of the A1 crystal (R1 =8.25 %) fully confirmed the model previously obtained for alacranite from the Uzon Caldera (Bonazzi et al., 2003b). Despite the smaller volume of the unit cell, all the sulfur positions were found to be fully occupied. It was then supposed that larger volumes could correspond to initial steps of light-induced alteration, which in molecular As sulfides usually produce an increase of the unit-cell volume due to the replacement of As4S4 (r-type) molecules with As4S4 (p-type) and As4S5 molecules. The effect of the light exposure on the structure of alacranite was thus investigated employing either a 550 or a 440 nm long wavelength pass filter (LWPF). A Schott KL 1500 electronic apparatus equipped with optical fibres and a 150 W FFR Sylvania dichroic halogen lamp was used to irradiate the sample with polychromatic light at a distance of 2.5 cm. This apparatus, also equipped with a visible pass filter, prevented infrared radiation from reaching the sample (IR transmittance ). The LWPF 550 and 440 nm long-wavelength pass filters (Schott Germany) were used to cut the region of the visible spectrum. The emission spectrum of the filtered light ranged from ∼500 to ∼800 nm, with an emission maximum at about 500/600 nm. The power density on the sample, measured with a power-meter instrument (model 362; Scientech, Boulder, Colorado), was about 70 mW cm−2.
Table 1Unit-cell values of alacranite (crystal A1) collected before and after exposure to filtered light.
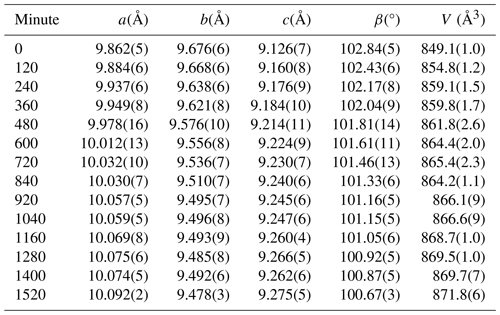
The action of the light filtered using a 550 nm LWPF did not produce any significant effect on the unit cell, thus confirming what was previously observed for alacranite from the type locality (Bonazzi et al., 2003b, 2006). On the other hand, employing the 440 nm LWPF, remarkable variations of the unit-cell parameters were observed, producing an increase of the unit-cell volume of 2.7 % after 1520 min of lightening (Table 1). In particular, the a and c parameters strongly increase, while the b parameter and the β angle decrease as a function of the light exposure time (Fig. 2). After 1520 min, a new diffraction experiment indicated that alacranite transformed into an orthorhombic phase (space group Pccn) identical to that already obtained by the light-induced alteration of a non-stoichiometric As4S4+x crystal (Bindi and Bonazzi, 2007).
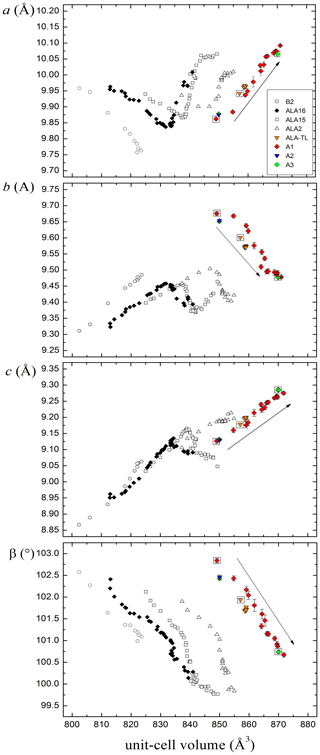
Figure 2Behaviour of alacranite crystals from Lăzăreşti (A1, A2, A3) compared with that of synthetic β-As4S4 phase (B2) and non-stoichiometric As8S9−x crystals from Radvanice (ALA16, ALA15, ALA2; Bonazzi et al., 2003a); orange triangles represent alacranite from the type locality exposed to 550 nm LWPF light (Bonazzi et al., 2006). Symbols inscribed within empty squares indicate samples where a structural study was conducted. Arrows indicate increasing exposure times.
To follow the evolution of the transformation path, a second crystal (A2; a=9.875(4), b=9.654(3), c=9.129(2) Å, β=102.46(5)°, V=850(8) Å3) was exposed to the 440 nm LWPF light for 900 min. However, when the crystal was examined by single-crystal X-ray diffraction, it resulted to be completely transformed into the orthorhombic Pccn phase. The subsequent structure refinement of the Pccn phase (R1 =12.05 %) confirmed the model previously obtained (Bindi and Bonazzi, 2007) and led to the chemical formula As4S4.93.
Finally, a third crystal was selected (A3; a=9.878(2), b=9.651(2), c=9.131(4) Å, β=102.43(4)°, V=850(7) Å3) and exposed to the 440 nm LWPF light for 600 min. At this step, the measured unit cell was a=10.065(6), b=9.477(3), c=9.285(4) Å, β=100.74(4)° and V=870(8) Å3. Single-crystal X-ray diffraction intensity data were collected and corrected for standard Lorentz and polarization factors with the CrysAlis RED package (Oxford Diffraction, 2006). The program ABSPACK in CrysAlis RED (Oxford Diffraction, 2006) was used for the absorption correction. A total of 888 unique reflections were collected. The analysis of the systematic absences together with the statistics tests on the distribution of values led to the choice of the space group . The structure was solved by direct methods and refined using the Shelx software package (Sheldrick, 2008) and Fourier syntheses. All atoms were located on Fo Fourier maps. Isotropic full-matrix least-squares cycles were initially run with the atom sites fully occupied, although the unusually high value of the isotropic displacement factor for most of the As and S atoms suggested partial occupancy at these sites. Successive least-squares cycles were done by fixing, alternately, the site-occupancy factor and the isotropic displacement parameter for these atoms. In the last cycle, partial occupancy factors were fixed to the refined values, and an anisotropic model of the whole structure was refined. Convergence was achieved at R=9.43 % for 468 observed reflections [Fo>4σ(Fo)] and 60 refined parameters. Scattering curves for neutral As and S atoms were taken from the International Tables for X-ray Crystallography (Ibers and Hamilton, 1974). Inspection of the difference Fourier map revealed that maximum positive and negative peaks were 0.43 and 0.64 e−/Å3, respectively. Fractional atomic coordinates and equivalent displacement parameters are given in Table 2.
The resulting structural model is strongly disordered wherein different kinds of As4Sn molecules replace each other.
The atomic coordinates of the new monoclinic structure (Table 2) show similarities with those of bonazziite, natural β-As4S4 (Bindi et al., 2015). The two As atoms of β-As4S4 are here split into five partially occupied sites. In detail, As1 of bonazziite splits into two half-occupied sites (As1a and As1b), whereas As2 splits into three sites (As2a, As2b and As2c). More complex is the scenario for the S atoms. In β-As4S4 there are three fully occupied S atoms. In the new monoclinic phase, only S1 remains fully occupied, S2 is partially vacant, S3 splits into two close partially occupied positions, and there is the appearance of a new, half-occupied sulfur atom (i.e. S4). All the atoms show very large atomic displacement parameters, indicating a strong disorder of the whole structure.
Taking into account the overall geometry and the partial occupancies of the structural sites, a complex model of interacting molecules was derived (Table 3). In detail, As1a links As2b, S1 and S3a, and As2b links As1a, As2b and S3a to form the As4S3 molecules (Fig. 3a). The As–S bond distances (with errors between 0.01 and 0.03 Å) are in the range of 2.30–2.37 Å and the As–As distances in the range 2.34–2.78 Å. Then, As1a links As2c, S1 and S3a, and As2c links As1a, S2 and S3a to form realgar-type As4S4 molecules (Fig. 3b). The As–S bond distances are in the range of 2.16–2.37 Å, and the As–As distance is 2.64 Å. As1b links S1, S3a and S4, and As2b links As2b, S3a and S4 to form uzonite-type As4S5 molecules (Fig. 3c). The As–S bond distances are in the range of 2.09–2.30 Å, and the As–As distance is 2.34 Å. Finally, As1b links S1, S3b and S4, and As2a links S2, S3b and S4 to form the new As4S6 molecule (Fig. 3d). The As–S bond distances are in the range of 2.00–2.32 Å.
Although some drawbacks are present due to the low quality of the available data given the complex material (few very short bond distances and large atomic displacement parameters), the overall sum of the atoms per formula unit assigned on the basis of the geometrical compatibility between split sites produces the formula As4S4.57. This is in excellent agreement with that obtained by the refinements of the occupancy factors (i.e. As4S4.60). The assignment of the different molecules is also corroborated by the almost perfect match between the calculated polyhedral volumes of the As4 disphenoidic group (2.77, 3.33 and 4.01 Å3 for the As4S3, As4S4 and As4S5, respectively) with those obtained for pure molecules (2.77, 3.23 and 4.38 Å3 for the As4S3, As4S4 and As4S5, respectively; Bonazzi and Bindi, 2008). The new As4S6 molecule exhibits a volume of 6.47 Å3, well beyond the largest value observed for the As4S5 molecule in the structure of wakabayashilite (4.89 Å3; Bonazzi et al., 2005).
Interestingly, the Fibonacci sequence is related to an incredible variety of unrelated, natural phenomena. Documented phyllotaxic relationships follow the numbering, and many examples of spirals in natura – such as those of Nautilus seashells or the arrangement of seeds on flower heads (e.g. sunflower) and pinecones – can be built on Fibonacci sequences (Mitchison, 1977). It could be a simple coincidence, but it is intriguing to see as the volume of the As4 group in the As4Sn () molecules exhibits a linear trend (r2=0.998) when plotted versus the range 1–21 with the known molecules falling exactly along the Fibonacci sequence (Fig. 4).
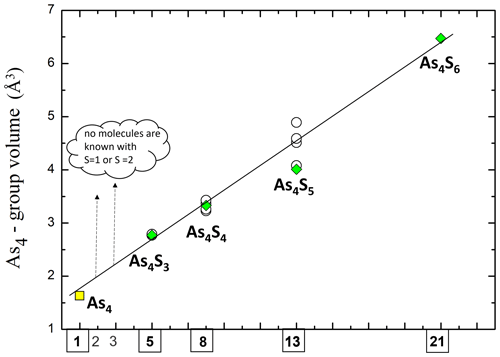
Figure 4Volume of the As4 group in the As4Sn () molecules exhibits a linear trend (r2=0.998) when plotted versus the range 1–21 of the Fibonacci sequence. Green diamonds denote this study (A3 crystal); empty circles denote data from literature (Bonazzi and Bindi, 2008); yellow square denotes theoretical volume of a tetrahedral As4 group having an As–As edge (corresponding to As–As covalent bond) of 2.40 Å.
As shown in Fig. 2, the unit-cell volume increases as a function of the exposure time to light. The expansion is observed for all the crystals (B2 (β-As4S4; Bonazzi et al., 1996); non-stoichiometric As8S9−x crystals (ALA16, ALA15, ALA2; Bonazzi et al., 2003a)) but ALA-TL (Bonazzi et al., 2003b), where only a minor increment was observed. As documented by Bonazzi et al. (2006), the volume increase is not linear with time, being greater at the beginning of the lightening experiment. For non-stoichiometric crystals (i.e. ALA16, ALA15 and ALA2), the cell increase shows a decreased rate occurring at diverse volume values (∼834, ∼842 and ∼851 Å3 for ALA16, ALA15 and ALA2, respectively) persisting for various exposure times. To compare the unit-cell values in different arsenic sulfides, the lattice parameters were plotted against the unit-cell volume rather than the time of lightening. The expansion and/or contraction of the unit-cell parameters in the monoclinic phase here discovered has a roughly constant trend. This is in contrast with what observed for non-stoichiometric As8S9−x crystals (ALA16, ALA15, ALA2). Bonazzi et al. (2006) individuated two paths: path I (beginning of the process) with a decrease of the a parameter and an increase of b and c and path II with an increase of the a parameter and a decrease of b and c. The monoclinic phase shows instead a single path with increasing a and c and decreasing the b parameter (path II only). A possible explanation for the diverse observed behaviour can be found in the different way in which the various molecules are arranged. Unfortunately, owing to the inherent limit of X-ray diffraction together with the extremely low diffraction quality of the crystals, we can only detect disordered mixtures of the various molecules without a clear hint of the possible arrangement mechanisms. Several reactions were hypothesized (Bonazzi et al., 2006), but the only clear evidence was the involvement of additional sulfur to the As4S4 and As4S5 molecules. In this study we have found the first direct evidence of the existence of the As4S6 molecule, which can well take into account the increase of the unit-cell volume in alacranite as well as the inversion from path I to path II observed by Bonazzi et al. (2006) for non-stoichiometric As8S9−x crystals. However, the results obtained in this study suggest that the extent of sulfur incorporation is strictly controlled by the type of molecular packing and not only by the kind of molecule. Indeed, it should be taken into consideration that the packing of molecules (see Bonazzi and Bindi, 2008) in the structure of bonazziite, β-As4S4 (Bindi et al., 2015), non-stoichiometric As4S4+x (Bonazzi et al., 2003a), alacranite (Bonazzi et al., 2003b), and the As4S5−x molecules in the orthorhombic Pccn phase (Bindi and Bonazzi, 2007) is substantially identical to the As4S4 molecules in the new monoclinic phase and to that of the As4S4 molecules in the structure of pararealgar (Bonazzi et al., 1995). In these transformations (β-As4S4→ pararealgar, and As4S5→ monoclinic phase), the final phase is produced by a continuous, room-temperature, light-induced alteration process which does not require a complete rearrangement of the molecular packing and therefore does not imply the loss of coherency between crystalline domains.
Over the past 40 years, many researchers have dealt with studies about structural modifications of arsenic sulfides belonging to the As8S8–As8S9 series when exposed to light with appropriate wavelength radiation. In this work, the photoinduced structural modifications of alacranite have been investigated by exposing the mineral to a 440 nm wavelength radiation (at room temperature) and studied by means of single-crystal X-ray diffraction. The results of this study allowed confirmation of the increase of the unit-cell volume as a function of the light exposure. Besides, it was possible to observe that, by increasing the light exposure to at least 600 min, there is a structural transition from alacranite sensu stricto (Bonazzi et al., 2003b), through an orthorhombic Pccn phase (Bindi and Bonazzi, 2007) to a new, monoclinic phase where four discrete molecular clusters (i.e. As4S3, As4S4, As4S5, As4S6) coexist and constantly evolve. Noteworthy is the presence of the As4S6 molecule, because it is the first time that such a molecule is reported in a crystalline compound of the As–S system.
This example conclusively corroborates the complexity of minerals in the natural kingdom, illustrating the intricate and multifaceted nature of their formation, composition and characteristics. This complexity underscores the rich diversity and sophistication inherent in Earth's geological processes (Bindi et al., 2020).
Crystallographic data (CCDC 2366909) can be obtained free of charge from The Cambridge Crystallographic Data Centre via https://doi.org/10.5517/ccdc.csd.cc2kfyxw (Bindi, 2024).
The study was conceived by LB and PB. LB, PB, LC, MMNF, GOL, MM and AT carried out the diffraction experiments. LB, PB, GP, MZ and SM discussed the results. LB wrote the paper. All the authors commented on the final version of the paper.
At least one of the (co-)authors is a member of the editorial board of European Journal of Mineralogy. The peer-review process was guided by an independent editor, and the authors also have no other competing interests to declare.
This article is part of the special issue “Celebrating the outstanding contribution of Paola Bonazzi to mineralogy”. It is not associated with a conference.
Publisher’s note: Copernicus Publications remains neutral with regard to jurisdictional claims made in the text, published maps, institutional affiliations, or any other geographical representation in this paper. While Copernicus Publications makes every effort to include appropriate place names, the final responsibility lies with the authors.
This article is in loving memory of Paola Bonazzi, who prematurely passed away before her time, whose passion for teaching illuminated countless minds, and whose kindness and dedication left an indelible mark on all who had the privilege of learning from her. Though gone too soon, she remains a guiding light in our academic journey, forever cherished and deeply missed. This work is dedicated to her, with gratitude and reverence for her enduring influence. The manuscript profited from the revision of Giovanni Ferraris and an anonymous reviewer as well as from the efficient editorial handling by Dmitry Pushcharovsky. Single-crystal X-ray diffraction studies were done at CRIST, Centro di Cristallografia Strutturale, University of Florence, Italy.
This research has been supported by Progetto di Ateneo 2023, University of Florence, issued to Luca Bindi.
This paper was edited by Dmitry Pushcharovsky and reviewed by Ferraris Giovanni and one anonymous referee.
Bindi, L.: CCDC 2366909: Experimental Crystal Structure Determination, The Cambridge Crystallographic Data Centre [data set], https://doi.org/10.5517/ccdc.csd.cc2kfyxw, 2024.
Bindi, L. and Bonazzi, P.: Light-induced alteration of arsenic sulfides: A new product with an orthorhombic crystal structure, Am. Mineral., 92, 617–620, 2007.
Bindi, L., Popova, V. I., and Bonazzi, P.: Uzonite, As4S5, from the type-locality: X-ray single crystal study and lighting experiments, Can. Mineral., 41, 1463–1468, 2003.
Bindi, L., Pratesi, G., Muniz-Miranda, M., Zoppi, M., Chelazzi, L., Lepore, G. O., and Menchetti, S.: From ancient pigments to modern optoelectronic applications of arsenic sulfides: bonazziite, the natural analogue of β-As4S4 from Khaidarkan deposit, Kyrgyzstan, Mineral. Mag., 79, 121–131, 2015.
Bindi, L., Nespolo, M., Krivovichev, S. V., Chapuis, G., and Biagioni, C.: Producing highly complicated materials. Nature does it better, Rep. Prog. Phys., 83, 106501–106540, 2020.
Bonazzi, P. and Bindi, L.: A crystallographic review of arsenic sulfides: Effects of chemical variations and changes induced by exposure to light, Z. Kristallogr., 223, 132–147, 2008.
Bonazzi, P., Menchetti, S., and Pratesi, G.: The crystal structure of pararealgar, As4S4, Am. Mineral., 80, 400–403, 1995.
Bonazzi, P., Menchetti, S., Pratesi, G., Muniz-Miranda, M., and Sbrana, G.: Light-induced variations in realgar and β-As4S4: X-ray diffraction and Raman studies, Am. Mineral., 81, 874–880, 1996.
Bonazzi, P., Bindi, L., Olmi, F., and Menchetti, S.: How many alacranites do exist? A structural study of non-stoichiometric As8S9−x crystals, Eur. J. Mineral., 15, 282–288, 2003a.
Bonazzi, P., Bindi, L., Popova, V.I., Pratesi, G., and Menchetti S.: Alacranite, As8S9: structural study of the holotype and re-assignment of the original chemical formula, Am. Mineral., 88, 1796–1800, 2003b.
Bonazzi, P., Lampronti, G.I., Bindi, L., and Zanardi, S.: Wakabayashilite, [(As,Sb)6S9][As4S5]: crystal structure, pseudosymmetry, twinning and revised chemical formula, Am. Mineral., 90, 1108–1114, 2005.
Bonazzi, P., Bindi, L., Pratesi, G., and Menchetti, S.: Light-induced changes in molecular arsenic sulfides: state of the art and new evidence by single-crystal X-ray diffraction, Am. Mineral., 91, 1323–1330, 2006.
Bonazzi, P., Bindi, L., Muniz Miranda, M., Chelazzi, L., Rödl, T., and Pfitzner, A.: Light-induced molecular change in synthetic HgI2⋅ As4S4: evidence by single-crystal X-ray diffraction and Raman spectroscopy, Am. Mineral., 96, 646–653, 2011.
Bonazzi, P., Lepore, G. O., and Bindi, L.: Molecular versus layered structure in arsenic sulphide minerals: the case of duranusite, As4S, Eur. J. Mineral., 28, 147–151, 2016.
Emelina, A. L., Alikhanian, A. S., Steblevskii, A. V., and Kolosov, E. N.: Phase diagram of the As-S system, Inorg. Chem., 43, 95–104, 2007.
Holomb, R., Mitsa, V., Johansson, P., Mateleshko, N., Matic, A., and Veresh, M.: Energy-dependence of light-induced changes in g-As45S55 during recording the micro-Raman spectra, Chalc. Lett., 2, 63–69, 2005.
Ibers, J. A. and Hamilton, W. C. (Eds.): International Tables for X-ray Crystallography, vol. IV, 366 pp., Kynock, Dordrecht, The Netherlands, 1974.
Kyono, A.: Ab initio quantum chemical investigation of arsenic sulfide molecular diversity from As4S6 and As4, Phys. Chem. Miner., 40, 717–731, 2013.
Kyono, A., Kimata, M., and Hatta, T.: Light-induced degradation dynamics in realgar: in situ structural investigation using single-crystal X-ray diffraction study and X-ray photoelectron spectroscopy, Am. Mineral., 90, 1563–1570, 2005.
Kristály, F., Szakáll, S., Bonazzi, P., Bindi, L., and Papucs, A.: Neogen volcanism related arsenic sulphide paragenesis from Lazaresti and Bodoc (Ciomadu Area, Harghita Mts.), and Covasna, Rom, J. Mineral Deposits and Rom. J. Mineral., 82, 192–195, 2006.
Mitchison, G. J.: Phyllotaxis and the Fibonacci series, Science, 196, 270–275, 1977.
Mullen, D. J. E. and Nowacki, W.: Refinement of the crystal structures of realgar, AsS and orpiment, As2S3, Z. Kristallogr., 136, 48–65, 1972.
Naumov, P., Makreski, P., and Jovanovski, G.: Direct atomic scale observation of linkage isomerization of As4S4 clusters during the photoinduced transition of realgar to pararealgar, Inorg. Chem., 46, 10624–10631, 2007.
Naumov, P., Makreski, P., Petruševski, G., Runčevski, T., and Jovanovski, G.: Visualization of a discrete solid-state process with steady-state X-ray diffraction: Observation of hopping of sulfur atoms in single crystals of realgar, J. Am. Chem. Soc., 132, 11398–11401, 2010.
Němec, P., Jedelský, J., Frumar, M., Černosěk, Z., and Vlček, M.: Structure of pulsed-laser deposited arsenic-rich As–S amorphous thin films, and effect of light and temperature, J. Non-Crystal. Solids, 351, 3497–3502, 2005.
Oxford Diffraction: CrysAlis RED (Version 1.171.31.2) and ABSPACK in CrysAlis RED. Oxford Diffraction Ltd, Abingdon, Oxfordshire, England, 2006.
Pratesi, G. and Zoppi, M.: An insight into the inverse transformation of realgar altered by light, Am. Mineral., 100, 1222–1229, 2015.
Sheldrick, G. M.: A short history of SHELX, Acta Crystallogr., A64, 112–122, 2008.
Trentelman, K., Stodulski, L., and Pavlosky, M.: Characterization of pararealgar and other light-induced transformation products from realgar by Raman microspectroscopy, Anal. Chem., 68, 1755–1761, 1996.
Vermeulen, M., Sanyova, J., and Janssens, K.: Identification of artificial orpiment in the interior decorations of the Japanese tower in Laeken, Brussels, Belgium, Heritage Sci., 3, 9, https://doi.org/10.1186/s40494-015-0040-7, 2015.
Zoppi, M. and Pratesi, G.: The dual behavior of the β-As4S4 altered by light, Am. Mineral., 97 890–896, 2012.
Zoppi, M., Bindi, L., Rödl, T., Pfitzner, A., and Bonazzi, P.: Light-induced structural changes in (HgBr2)3(As4S4)2: An X-ray single-crystal diffraction, Raman spectroscopy and ab initio study, Solid State Sci., 23, 88–95, 2013.