the Creative Commons Attribution 4.0 License.
the Creative Commons Attribution 4.0 License.
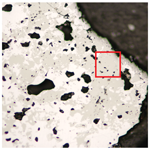
Tetrahedrite-(Cd), Cu6(Cu4Cd2)Sb4S13, from Radětice near Příbram, Czech Republic: the new Cd member of the tetrahedrite group
Jiří Sejkora
Cristian Biagioni
Pavel Škácha
Silvia Musetti
Anatoly V. Kasatkin
Fabrizio Nestola
Tetrahedrite-(Cd), Cu6(Cu4Cd2)Sb4S13, is a new IMA-approved mineral species from Radětice near the Příbram deposit, Czech Republic. It occurs as black metallic anhedral grains, up to 200 µm in size in quartz–calcite gangue, associated with galena, bournonite, sphalerite, pyrite, geocronite, silver, stromeyerite, tetrahedrite-(Zn), tetrahedrite-(Fe), tetrahedrite-(Cu), and an unknown Pb-rich tetrahedrite-like phase. In reflected light, tetrahedrite-(Cd) is isotropic and grey with greenish tints. Reflectance data for the four COM wavelengths in air are as follows (λ (nm) : R (%)): 470 : 30.8, 546 : 31.1, 589 : 31.1 and 650 : 28.8. Electron microprobe analysis gave (in wt % – average of 13 spot analyses) Cu 34.85, Ag 2.09, Fe 0.18, Zn 0.26, Cd 11.03, Hg 0.75, Pb 0.31, Sb 28.07, and S 23.38, with a total of 100.92. On the basis of ΣMe = 16 atoms per formula unit, the empirical formula of tetrahedrite-(Cd) is Cu9.65Ag0.34Cd1.73Zn0.07Hg0.07Fe0.06Pb0.03Sb4.06S12.84. Tennantite-(Cd) is cubic, , with unit-cell parameters a=10.504(3) Å, V=1158.9(9) Å3, and Z=2. Its crystal structure was refined by single-crystal X-ray diffraction data to a final R1=0.0252 on the basis of 257 unique reflections with Fo>4σ(Fo) and 23 refined parameters. Tetrahedrite-(Cd) is isotypic with other tetrahedrite-group minerals. Its crystal chemistry is discussed, and previous findings of Cd-rich tetrahedrite-group minerals are briefly reviewed.
- Article
(1259 KB) - Full-text XML
-
Supplement
(135 KB) - BibTeX
- EndNote
Tetrahedrite-group minerals are the most common sulfosalts in different kinds of hydrothermal ore deposits. They form a complex isotypic series, with the general structural formula , where A = Cu+, Ag+, and □ (vacancy); B = Cu+ and Ag+; C = Zn2+, Fe2+, Hg2+, Cd2+, Ni2+, Mn2+, Cu2+, Cu+, In3+, and Fe3+; D = Sb3+, As3+, Bi3+, and Te4+; Y = S2−, and Se2−; and Z = S2−, Se2−, and □ (Biagioni et al., 2020a). Thus, tetrahedrite-group minerals are characterized by different homo- and heterovalent substitutions. The nomenclature of the tetrahedrite-group minerals was recently proposed by Biagioni et al. (2020a), also taking into account the different C chemical constituents, usually represented by divalent transition elements, for classification purposes.
The Cd-bearing tetrahedrite-group minerals are generally rare, but some Cd-dominant chemical compositions have been reported previously (see a brief review below). Recently, three Cd-dominant members of the tetrahedrite group were defined: tennantite-(Cd) from Berenguela, Bolivia (Biagioni et al., 2022a); hakite-(Cd) from Příbram, Czech Republic (Sejkora et al., 2022); and argentotetrahedrite-(Cd) from Rudno nad Hronom, Slovakia (Mikuš et al., 2023). A systematic investigation of tetrahedrite-group minerals from the Příbram uranium and base-metal ore district led to the recognition of a new potential Cd member of the tetrahedrite series. Further chemical and crystallographic investigations confirmed such a preliminary identification, allowing the proposal of the new mineral species tetrahedrite-(Cd).
The new mineral and its name, in keeping with the current nomenclature of tetrahedrite group (Biagioni et al., 2020a), have been approved by the Commission on New Minerals, Nomenclature and Classification of the International Mineralogical Association, under the voting number 2022-115. Its mineral symbol, in accord with Warr (2021), is Ttr-Cd. Holotype material (polished section) of tetrahedrite-(Cd) is deposited in the collections of the Department of Mineralogy and Petrology, National Museum in Prague, Cirkusová 1740, 193 00 Prague 9, Czech Republic, under the catalogue number P1P 43/2022, whereas the grain used for single-crystal X-ray diffraction study is kept in the collections of the Museo di Storia Naturale of the Università di Pisa, Via Roma 79, Calci (PI), under catalogue number 20025.
Tetrahedrite-(Cd) has been found in material mined from the vein S-1 in the area of the seventh and eighth levels of the Radětice shaft (GPS coordinates: 49∘38′20.44′′ N, 14∘05′13.66′′ E) located about 300 m east of the village of Radětice, 5 km southeast of Příbram, central Bohemia, Czech Republic. The Radětice deposit is one of the smaller base-metal and uranium deposits in the outer part of the Příbram ore area. Small-scale mining operations for prevailing iron and minor lead ores took place in several periods in the Hallstatt age (600–500 BCE) and in the 13th–15th and 19th centuries in the area of Radětice. The geochemical research connected with the extensive mining operations in the Příbram uranium and base-metal district proceeded in the Radětice area in 1969, and a large Ag anomaly was found during that operation. Subsequently, the Radětice shaft was built in 1970 and reached a final depth of 462 m in 1978. Small-scale but rich Ag(+Pb, Zn) ore mineralization was found between the 6th and 10th levels, where 1372 t of ore was mined between the 7th and 8th levels (Štekl et al., 1984b).
The Radětice deposit is hosted in the Carboniferous rocks of the central Bohemian plutonic complex, around 5 km from the contact with upper Proterozoic rocks of the Barrandian sedimentary unit in which the most significant base-metal Březové Hory ore district and base-metal and uranium Příbram ore district are located. Several types of magmatic rocks occur in the Radětice deposit. The oldest ones are represented by gabbro and dark quartz diorite, followed by middle-grained amphibole–biotite quartz diorite and granodiorite. The youngest magmatic rocks are coarse-grained granodiorite to granite and finally aplite (Štekl et al., 1984a). Tectonically the area is characterized by the Stěžov fault belt. The northeastern branch of this belt was named the S-2 vein, where only insignificant Pb–Zn ore mineralization was found. The southeastern branch is the vein S-1, where the Ag–Pb ore mineralization was found. The direction of this vein is 310∘, and its dip is mostly steep, 85∘ to the NW. The ore-mineralized body is 300 m long in the eighth level, and its vertical dimension does not exceed 240 m (Štekl et al., 1981). The most significant ore mineralization is tied to a NW–SE direction, which is common with the nearby Vrančice deposit showing a similar geology and mineralogy (Blüml et al., 1984).
The mineralogy of the vein S-1 is rather homogeneous, being formed by quartz and (Pb–Mn)-rich calcite accompanied by iron oxides and hydroxides. According to Štekl et al. (1984b), galena, sphalerite, tetrahedrite-group minerals, siderite, hematite, and goethite formed during a second stage of mineralization, whereas the occurrence of native silver with stromeyerite, and rarer acanthite, bornite, and galena, associated with quartz and (Pb–Mn)-rich calcite, is due to a third mineralization stage. The ore-mineralized part of the S-1 vein is developed in coarse-grained granodiorite and ends up on the contact with diorite, where the vein fades into several thin veinlets. Tetrahedrite-(Cd) occurs in quartz–calcite gangue in close association with galena, bournonite, sphalerite, pyrite, geocronite, native silver, stromeyerite, tetrahedrite-(Zn), tetrahedrite-(Fe), Pb- and Cd-rich tetrahedrite-(Cu), and an unknown Pb-rich tetrahedrite-like phase (under investigation). The crystallization of tetrahedrite-(Cd) is related to a low-T hydrothermal event.
Recently, tetrahedrite-(Cd) was also identified during the reinvestigation of an old museum sample from the historical Zmeinogorsk Mine (Altai Krai, Western Siberia, Russia). This historical mine was discovered in 1725 as a rich deposit of copper ore, but underground workings developed mostly for silver and gold. In the 20th century it was mostly mined for Zn–Pb–Cu ores until 1968, when industrial work completely stopped due to its unprofitability. The history, geology, and mineralogy of the Zmeinogorsk Mine can be found elsewhere (e.g. Timofeevskiy, 1940; Garmash, 1960; Bestemyanova and Grinev, 2021). The sample containing the new mineral was collected in 1930, and since then it has been stored in the Vernadsky Geological Museum in Moscow (Russia), under the catalogue number MH-00683 and accompanied by the label “gold, argentite, sternbergite”. Tetrahedrite-(Cd) forms areas of up to 0.05 × 0.05 mm in tetrahedrite-(Zn), associated with acanthite, covellite, cupropolybasite, galena, gold, imiterite, mckinstryite, sphalerite, stromeyerite, tetrahedrite-(Hg), and tetrahedrite-(Mn).
In holotype material, tetrahedrite-(Cd) occurs as anhedral grains, up to 200 µm in size (Fig. 1) and black in colour, with a black streak and metallic lustre. Mohs hardness was not measured, but it should be close to 4, in agreement with other members of the tetrahedrite group. Tetrahedrite-(Cd) is brittle, with a conchoidal fracture and indistinct cleavage. Owing to the small amount of available material, density was not measured; based on the empirical formula and unit-cell parameters derived from single-crystal X-ray diffraction, the calculated density is 5.094 g cm−3. In reflected light, tetrahedrite-(Cd) is isotropic. It is grey, with greenish tints (Radětice) or greenish–brownish tints (Zmeinogorsk). Internal reflections were not observed. Reflectance values (Table 1) were measured in air using a Tidas MSP400 spectrophotometer and Leica microscope, with a 50× objective and Zeiss 370 WTiC standard (Radětice material) and an MSF-R microspectrophotometer with 50× objective and Si standard (Zmeinogorsk material). The reflectance curves for tetrahedrite-(Cd) from Radětice and Zmeinogorsk are very similar (Fig. 2). Figure 3 compares measured data with published reflectance curves for other tetrahedrite-series minerals and Cd-dominant members, respectively.
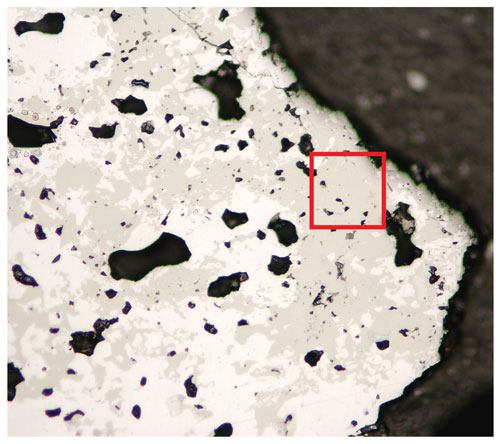
Figure 1Reflected-light image of tetrahedrite-(Cd) (grey) associated with galena (light), holotype sample. The grain studied through single-crystal X-ray diffraction was extracted from the red box. Field of view: 0.75 mm.
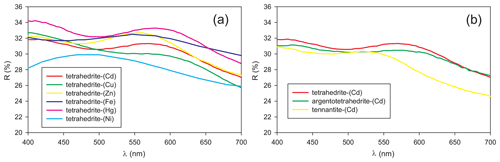
Figure 3Reflectance curve for tetrahedrite-(Cd) from the Radětice deposit, compared with published data for (a) other tetrahedrite-series minerals – tetrahedrite-(Cu), Bankov deposit, Slovakia (Sejkora et al., 2023); tetrahedrite-(Zn), Le Freney-d'Oisans, Isère, France (Criddle and Stanley, 1993); tetrahedrite-(Fe), Frigido Mine, Massa, Tuscany, Italy (Criddle and Stanley, 1993); tetrahedrite-(Hg), Buca della Vena Mine, Apuan Alps, Tuscany, Italy (Biagioni et al., 2020b); and tetrahedrite-(Ni), Luobusa, Tibet, China (Wang et al., 2023) – and (b) Cd-dominant tetrahedrite-group minerals – argentotetrahedrite-(Cd), Rudno nad Hronom, Slovakia (Mikuš et al., 2023), and tennantite-(Cd), Berenguela, Bolivia (Biagioni et al., 2022a).
Quantitative chemical analyses on type material from Radětice were carried out using a Cameca SX100 electron microprobe (National Museum of Prague, Czech Republic) and the following experimental conditions: WDS mode, accelerating voltage 25 kV, beam current 20 nA, and beam diameter 1 µm. Standards (element, emission line) were Ag (AgLα), CdTe (CdLα), chalcopyrite (CuKα, SKα), HgTe (HgLα), PbS (PbMα), pyrite (FeKα), Sb2S3 (SbLα), and ZnS (ZnKα). Contents of other elements with atomic numbers >8 are below detection limits (including As, Te, and Se).
Quantitative chemical data on the sample from the Zmeinogorsk Mine were collected using a Tescan Solaris FEG-SEM equipped with an Oxford Instruments Wave 700 WDS (Dipartimento di Geoscienze, Università di Padova, Italy). Experimental conditions were as follows: WDS mode, accelerating voltage 25 kV, beam current 10 nA, and beam diameter 2 µm. Standards (element, emission line) were Ag (AgLα), Cu (CuKα), pyrite (FeKα, SKα), Cd (CdLα), GaAs (AsLα), MnTiO3 (MnKα), Sb (SbLα), and ZnS (ZnKα).
In both cases, matrix correction by PAP procedure (Pouchou and Pichoir, 1985) was applied to the data. Results are given in Table 2.
Powder X-ray diffraction data could not be collected owing to the small amount of the available material. Consequently, these data, given in Table 3, were calculated through the software PowderCell 2.3 (Kraus and Nolze, 1996) using the structural model of the sample from Radětice discussed below.
Table 3Calculated X-ray powder diffraction data for tetrahedrite-(Cd).
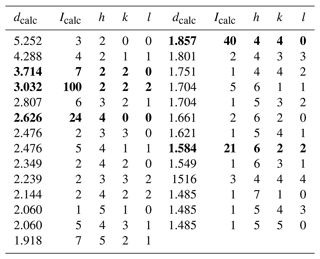
Relative intensity and dhkl (Å) were calculated using the software PowderCell 2.3 (Kraus and Nolze, 1996) on the basis of the structural model given in Tables 4 and 5. Only reflections with Icalc>1 are listed. The five strongest reflections are given in bold.
Single-crystal X-ray diffraction intensity data were collected using a Bruker D8 Venture four-circle diffractometer equipped with an air-cooled Photon III detector and microfocus MoKα radiation (Centro per l'Integrazione della Strumentazione Scientifica, Università di Pisa, Italy). The detector-to-crystal distance was set to 38 mm. Data were collected using φ scan modes, in 0.5∘ slices, with an exposure time of 15 s per frame. A total of 516 frames were collected, and frames were integrated with the Bruker SAINT software package using a narrow-frame algorithm. Data were corrected for Lorentz–polarization, absorption, and background. Unit-cell parameters were refined on the basis of the XYZ centroids of 1040 reflections above 20σI with 7.759<2θ<54.95∘ as a=10.504(3) Å, V=1158.9(9) Å3, and space group .
The crystal structure of tetrahedrite-(Cd) was refined using SHELXL 2018 (Sheldrick, 2015) starting from the atomic coordinates of Johnson and Burnham (1985). The occurrence of racemic twinning was modelled. The M(2) site was found to be split into two sub-positions, M(2a) and M(2b). The following neutral scattering curves, taken from the International Tables for Crystallography (Wilson, 1992), were used: Cu at M(2) (the sum of the site occupancies at the split positions was constrained to be 1), Cu vs. Cd at M(1), Sb at X(3), and S at the S(1) and S(2) sites. The anisotropic structural model converged to R1=0.0252 for 257 reflections with Fo>4σ(Fo) and 23 refined parameters. Details of data collection and refinement are given in Table 4. Fractional atomic coordinates and equivalent isotropic displacement parameters are reported in Table 5, along with a comparison between refined mean atomic numbers (MANs) and the values calculated on the basis of the site occupancies discussed below. Table 6 reports selected bond distances. Table 7 gives the weighted bond-valence calculations obtained using the bond-valence parameters of Brese and O'Keeffe (1991). A crystallographic information file (CIF) is deposited in the Supplement.
Table 5Site, site occupancy (s.o.), fractional atom coordinates, equivalent isotropic displacement parameters (Å2), and observed and calculated (from chemical data) mean atomic number (MANobs and MANcal, respectively) for tetrahedrite-(Cd).
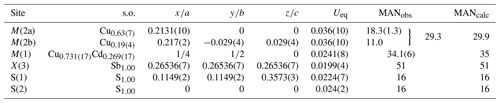
Note: MAN at M(2b) was calculated taking into account that this split position has a multiplicity twice that of M(2a).
5.1 Chemical formula
As discussed in previous papers (e.g. Sejkora et al., 2021), there are different approaches to recalculating the chemical formulae of tetrahedrite-group minerals. The two better ones normalize the number of atoms on the basis of ΣMe = 16 atoms per formula unit (apfu) or on the basis of (As + Sb + Te + Bi) = 4 apfu. The former approach assumes that no vacancies occur at the M(2), M(1), and X(3) sites, whereas the latter is mainly based on the results discussed by Johnson et al. (1986), who revealed that negligible variations in the ideal number of X(3) atoms usually occur.
The first approach gives the chemical formulae Cu9.65(4)Ag0.34(7)Cd1.73(7)Zn0.07(4)Hg0.07(2) Fe0.06(2)Pb0.03(2)Sb4.06(3)S12.84(10) (Radětice) and Cu9.59(12)Ag1.12(14)Cd1.04(19)Mn0.26(11)Zn0.10(5)Fe0.04(3)Sb3.25(17)As0.61(33)S12.95(62) (Zmeinogorsk), whereas the other normalization strategy corresponds to the formulae Cu9.52(4)Ag0.34(7)Cd1.70(7)Zn0.07(3)Hg0.06(2)Fe0.06(2)Pb0.03(2)Sb4.00S12.65(10) (Radětice) and Cu9.97(57)Ag1.16(16)Cd1.08(24)Mn0.26(11)Zn0.10(6)Fe0.04(4)Sb3.38(31)As0.62(31)S13.48(1.19) (Zmeinogorsk).
In the holotype sample from Radětice, the (Cu + Ag) content is very close to the ideal one, i.e. 10.00 apfu, ranging between 9.94 and 10.05 apfu (based on ΣMe = 16 apfu). is 0.034, ranging between 0.026 and 0.049. The C constituent is represented by dominant Cd (1.64–1.84 apfu) accompanied by minor Zn, Fe, and Hg, with Fe likely occurring as Fe3+, in agreement with previous authors (e.g. Makovicky et al., 1990, 2003; Nasonova et al., 2016). Minor Pb (up to 0.08 apfu) is here considered a C constituent, even if its actual structural role in tetrahedrite-group minerals is currently unknown. Arsenic was not detected in the sample from Radětice, which is consequently a pure Sb member.
Tetrahedrite-(Cd) from Zmeinogorsk displays a (Cu + Ag) content of 10.7 apfu and an average sum of (Cd + Mn + Zn + Fe) of 1.44 apfu, thus suggesting that some formally divalent Cu should occur as C constituent. The Russian sample is richer in Ag than the Czech one, having an ratio of 0.104, ranging between 0.090 and 0.115. Manganese occurs in relatively high concentrations (up to 0.35 apfu, on the basis of 16 Me apfu), in agreement with the results of Makovicky and Karup-Møller (1994) and the description of tetrahedrite-(Mn) by Momma et al. (2022). The atomic ratio is variable between 0.055 and 0.233, with an average value of 0.154.
The simplified formula of tetrahedrite-(Cd) is (Cu,Ag)6[Cu4(Cd,Zn,Hg,Fe)2]Sb4S13, corresponding to the end-member formula Cu6(Cu4Cd2)Sb4S13. It corresponds to (in wt %) Cu 36.02, Cd 12.74, Sb 27.61, and S 23.63, totalling 100.00.
5.2 Crystal structure description
Tetrahedrite-(Cd) is isotypic with the other members of the tetrahedrite group, whose general structural formula is M(2)6M(1)6X(3)4S(1)12S(2) (Biagioni et al., 2020a).
The electron density at the M(2) site was found to be split into two sub-positions, namely M(2a) and M(2b), separated by 0.43(6) Å, whereas the distance between two neighbouring M(2b) positions is 0.85(13) Å. These distances are shorter than those observed in other tetrahedrite-group minerals characterized by a split M(2) site (e.g. Cu-rich unsubstituted tennantite of Makovicky et al., 2005) and can be compared to those reported by Biagioni et al. (2022b) in tennantite-(Cu), i.e. 0.60(11) and 1.20(2) Å for M(2a)–M(2b) and M(2b)–M(2b) distances, respectively. The M(2a) site has a triangular planar coordination, whereas the M(2b) position has a flat trigonal pyramidal coordination. This feature agrees with previous studies (e.g. Andreasen et al., 2008; Welch et al., 2018; Biagioni et al., 2021). Average Me–S distances are 2.268 and 2.302 Å for M(2a) and M(2b), respectively (Table 6). The MAN refined at M(2a) + M(2b) is 29.3 electrons, to be compared with the MAN calculated on the basis of the site occupancy M(2)(Cu0.95Ag0.05), i.e. 29.9 electrons (e−) (Table 5). The bond-valence sum was calculated assuming that minor Ag was hosted at the larger M(2b) position. The bond-valence sum at M(2a) + M(2b) is 1.01 valence units (v.u.), in agreement with the proposed occupancy (Table 7).
The tetrahedrally coordinated M(1) site has an average Me–S distance of 2.391 Å (Table 6), longer than that observed in mixed (Cu,Zn,Fe) tetrahedrally coordinated sites in tetrahedrite-group minerals (e.g. 2.342 Å – Wuensch, 1964; 2.337 Å – Wuensch et al., 1966) and comparable to those observed in tennantite-(Cd) (2.370 Å – Biagioni et al., 2022a) and in argentotetrahedrite-(Cd) (2.38 Å - Mikuš et al., 2023). On the basis of the electron microprobe data, the occupancy at the M(1) site of tetrahedrite-(Cd) can be given as (CuCdZnHgFe). The mean atomic number at this site is 34.1 e− per site, to be compared with 35.0 e− calculated from the chemical data (Table 5). The bond-valence sum, 1.60 v.u., is higher than the theoretical value, 1.33 v.u. (Table 7). Such an overbonding of the M(1) site has been observed in other tetrahedrite-group minerals, e.g. tennantite-(Hg) (1.68 v.u.), tetrahedrite-(Hg) (1.64 v.u.), and tennantite-(Cd) (1.64 v.u.) (Biagioni et al., 2020b, 2021, 2022a). This discrepancy is probably due to the use of inappropriate bond parameters for Cd–S bonds (and Hg–S as well) and the mixed ionic covalent character of the Me–S bonds in tetrahedrite-group species (e.g. Weller and Morelli, 2022). Indeed, the observed average <M(1)–S(1) > distance is shorter than that calculated using ideal bond valence and bond valence parameters of Brese and O'Keeffe (1991): in detail, for tetrahedral coordination, the ideal Me–S bond distances should be the following: Cu+ 2.373 Å, Cd2+ 2.546 Å, Zn 2.346 Å, Hg 2.576 Å, and Fe3+ 2.266 Å. Assuming the site occupancy at M(1) proposed above, the calculated <M(1)–S(1) > distance would be 2.424 Å, longer than the observed one. Such a discrepancy was reported by Biagioni et al. (2022a) for tennantite-(Cd), and also using the Cd–S distance observed in greenockite (2.528 Å – Xu and Ching, 1993) and hawleyite (2.526 Å – Skinner, 1961) the calculated <M(1)–S(1) > distance still remains slightly longer (i.e. 2.418 Å, using an average value of Cd–S between the values reported in greenockite and hawleyite). Charnock et al. (1989), in their EXAFS study confirming the tetrahedral coordination of Cd in tetrahedrite-group minerals, reported a first S shell at 2.48 Å. Using such an observed Cd–S distance, the calculated value for the studied sample of tetrahedrite-(Cd) would be 2.405 Å, closer to the observed value. Finally, Johnson et al. (1988) gave a crystal radius for IVCd2+ of 0.84 Å, the same as IVHg2+. Indeed, the average <M(1)–S(1) > distance observed in tetrahedrite-(Cd) can be compared with those reported in tetrahedrite-(Hg) (2.3913 Å, with site occupancy Cu0.66Hg0.28Zn0.05Fe0.01; Biagioni et al., 2020b) and tennantite-(Hg) (2.389 Å, with site occupancy Cu0.73Hg0.27; Biagioni et al., 2021). Structural data of synthetic Cu10Cd2Sb4S13 are reported in the ICSD database (Allen et al., 1987). These data are based on unit-cell parameters given by Johnson (1991) and were refined on the basis of powder X-ray diffraction data. In this structure (please note that Cd is wrongly attributed to the M(2) site), the <M(1)–S(1) > distance is 2.367 Å, shorter than that observed in tetrahedrite-(Cd).
The X(3) site has an average bond distance of 2.434 Å (Table 6), and it is occupied by Sb only. The bond-valence sum, 3.12 v.u., agrees with such an occupancy (Table 7).
Finally, both the S(1) and the S(2) sites were found to be fully occupied by S. The bond-valence sums at the S(1) and S(2) sites are 2.17 and 2.10 v.u., respectively (Table 7).
5.3 Relation between Cd content and unit-cell parameter
The a unit-cell parameter of tetrahedrite-(Cd) is ∼ 10.50 Å. The increase in a with respect to ideal tetrahedrite-(Zn)/tetrahedrite-(Fe) (∼ 10.38 Å) is due to the occurrence of Cd2+, in agreement with Charlat and Lévy (1975), who observed the negligible role of the Zn–Fe substitution in affecting the unit-cell parameter of tetrahedrite-group minerals. Pattrick and Hall (1983) observed an increase of 0.06 Å per atom Cd replacing (Zn,Fe) in synthetic tetrahedrite. Consequently, on the basis of the assumed chemistry of type material of tetrahedrite-(Cd), the expected unit-cell parameter of the studied sample should be a ∼ 10.48 Å; moreover, taking into account the occurrence of minor Hg, having a similar size to Cd, a best estimate of the unit-cell parameter may be a∼10.49 Å, in accord with the observed value.
Johnson et al. (1987) proposed the following relation between chemistry and unit-cell parameters (for synthetic tetrahedrites, since no data were available for natural Cd-bearing tetrahedrites): a (Å) = 10.381 + 0.039 Ag + 0.003 Ag2 – 0.019 Cu* + 0.064 Cd – 0.037 As, where Cu* = [2 − (Fe + Zn + Hg + Cd)]. The calculated unit-cell parameter is a=10.493 Å, in keeping with the observed value (please take into account that minor Hg was neglected). The calculated unit-cell parameter, assuming the idealized chemical formula Cu6(Cu4Cd2)Sb4S13, is a=10.51 Å.
5.4 Cadmium in tetrahedrite-group minerals
Currently, six members of the tetrahedrite series have been reported: tetrahedrite-(Fe), tetrahedrite-(Zn), tetrahedrite-(Hg), tetrahedrite-(Mn), tetrahedrite-(Ni), and tetrahedrite-(Cu) (Biagioni et al., 2020a, b; Momma et al., 2022; Wang et al., 2023; Sejkora et al., 2023). Tetrahedrite-(Cd) is a further addition to this series. As discussed in Biagioni et al. (2022a), the rarity of Cd-bearing tetrahedrite-group minerals can be related not only to the low abundance of Cd in crustal rocks (0.102 µg g−1 in the upper continental crust – Wedepohl, 1995) but also to the preference of Cd to be partitioned into co-crystallizing sphalerite and chalcopyrite as suggested by George et al. (2017). In fact, these authors pointed out that Cd contents are higher in those tetrahedrite-group mineral samples crystallizing in sphalerite- and chalcopyrite-free assemblages or in unusually Cd-enriched environments.
Four members of the tetrahedrite group are characterized by Cd as dominant C constituents. In addition to tetrahedrite-(Cd), two other species are represented by the As-isotype tennantite-(Cd) and the Ag-isotype argentotetrahedrite-(Cd). The former was described from the Cd-rich hydrothermal veins of the Berenguela district, Bolivia (Biagioni et al., 2022a), whereas the latter is a very recent addition to the freibergite series and was reported from the Au–Ag epithermal deposits of Rudno nad Hronom, Slovakia (Mikuš et al., 2023). Finally, the selenide hakite-(Cd) was reported from Příbram, central Bohemia, Czech Republic (Škácha et al., 2016, 2017; Sejkora et al., 2022).
A survey of the available literature reveals that these species have been reported since the end of the 1970s. Tetrahedrite-(Cd) was reported by Pattrick (1978) from Tyndrum (Perthshire, Scotland, UK), along with potential argentotetrahedrite-(Cd). An intermediate member between this latter freibergite-series mineral and tetrahedrite-(Cd) was reported from the Ushkatyn-III deposit (Kazakhstan) by Voropaev et al. (1988) and by Jia et al. (1988) from Xitieshan (China). A mineral phase with composition corresponding to argentotetrahedrite-(Cd) was mentioned by Repstock et al. (2016) from the Mavrokoryfi deposit (Greece). Dobbe (1992) gave chemical data corresponding to composition varying between argentotetrahedrite-(Fe), “argentotetrahedrite-(Mn)”, and argentotetrahedrite-(Cd). Finally, to the best of our knowledge, the maximum Cd content in a tetrahedrite-group mineral is probably that reported by Voudouris et al. (2011) in tetrahedrite-(Cd) from Evia Island, Greece, i.e. 1.97 apfu.
The new life of the tetrahedrite-group minerals following the revision of their nomenclature (Biagioni et al., 2020a) has not simply allowed the definition of several new mineral species characterized, in some cases, by unusual end-member composition; more importantly, through the collection of modern (and usually high-quality) data, it has promoted a better understanding of the complex crystal chemistry of this isotypic series of sulfosalts. Cadmium-dominant members are among the latest addition, and, notwithstanding their exceedingly rarity, their identification could have different implications.
Indeed, tetrahedrite-group minerals are among the most widespread accessory sulfosalts in hydrothermal ore deposits, where sometimes they could host economically valuable elements or environmentally hazardous chemical constituents. In this respect, recently some studies have focused on the weathering of these chalcogenides (e.g. Borčinová Radková et al., 2017; Majzlan et al., 2018; Keim et al., 2018); since Cd is a potentially toxic element (e.g. Genchi et al., 2020), the occurrence of Cd-dominant species, among them tetrahedrite-(Cd), could potentially have environmental implications. According to Mikuš et al. (2023), the low natural abundances of some of these phases (e.g. argentotetrahedrite-(Cd)) suggest that is difficult to expect serious environmental issues related to such rare species. However, this is a possibility that should not be neglected, in particular in Cd-rich hydrothermal veins.
Moreover, tetrahedrite-group minerals are currently actively studied for their high-tech properties (e.g. Suekuni et al., 2014; Chetty et al., 2015; Levinsky et al., 2019). Among the chemical compositions showing interesting properties, synthetic Cu10Cd2Sb4S13 has potential electronic properties and for this reason has been the focus of several studies in the last decade (e.g. Kumar et al., 2016; Krustok et al., 2020; López Cota et al., 2021; Ghisani et al., 2020, 2022).
Crystallographic data for tetrahedrite-(Cd) are available in the Supplement.
The supplement related to this article is available online at: https://doi.org/10.5194/ejm-35-897-2023-supplement.
JS: conception of the project, EPMA and optical measurements, data interpretation, and writing of the paper with inputs from other authors. CB and SM: single-crystal X-ray measurements and data interpretation. PŠ: locality research and preparation of samples. AVK and FN: optical and EPMA measurements of sample from Zmeinogorsk.
At least one of the (co-)authors is a member of the editorial board of European Journal of Mineralogy. The peer-review process was guided by an independent editor, and the authors also have no other competing interests to declare.
Publisher's note: Copernicus Publications remains neutral with regard to jurisdictional claims made in the text, published maps, institutional affiliations, or any other geographical representation in this paper. While Copernicus Publications makes every effort to include appropriate place names, the final responsibility lies with the authors.
This article is part of the special issue “New minerals: EJM support”. It is not associated with a conference.
We appreciate the many constructive comments of reviewers Luca Bindi and Fernando Cámara that helped to improve this paper significantly. The Centro per l'Integrazione della Strumentazione scientifica dell'Università di Pisa (CISUP) is thanked for providing access to their single-crystal X-ray diffraction laboratory.
Jiří Sejkora and Pavel Škácha were supported by the Ministry of Culture of the Czech Republic (long-term project DKRVO 2019-2023/1.II.e; National Museum, 00023272) and the Czech Science Foundation (project 19-16218S). Cristian Biagioni was supported by the Ministero dell'Istruzione, dell'Università e della Ricerca through the project PRIN 2017 “TEOREM – deciphering geological processes using Terrestrial and Extraterrestrial ORE Minerals”, prot. 2017AK8C32.
This paper was edited by Cristiano Ferraris and reviewed by Luca Bindi and Fernando Cámara.
Allen, F. H., Bergerhoff, G., and Sievers, R.: Crystallographic Databases, International Union of Crystallography, Chester, England, 221 pp., 1987.
Andreasen, J. W., Makovicky, E., Lebech, B., and Karup-Møller, S.: The role of iron in tetrahedrite and tennantite determined by Rietveld refinement of neutron powder diffraction data, Phys. Chem. Miner., 35, 447–454, 2008.
Bestemyanova, K. V. and Grinev, O. M.: Mineralogy of baryte-polymetallic deposits of Zmeinogorskiy ore district (Rudnyi Altai), Bulletin of the Tomsk Polytechnic University. Engineering of Georesources, 332, 210–222, 2021 (in Russian).
Biagioni, C., George, L. G., Cook, N. J., Makovicky, E., Moëlo, Y., Pasero, M., Sejkora, J., Stanley, C. J., Welch, M. D., and Bosi, F.: The tetrahedrite group: Nomenclature and classification, Am. Miner., 105, 109–122, 2020a.
Biagioni, C., Sejkora, J., Musetti, S., Velebil, D., and Pasero, M.: Tetrahedrite-(Hg), a new “old” member of the tetrahedrite group, Mineral. Mag., 84, 584–592, 2020b.
Biagioni, C., Sejkora, J., Raber, T., Roth, P., Moëlo, Y., and Dolníček, Z.: Tennantite-(Hg), a new tetrahedrite group mineral from the Lengenbach quarry (Binn, Switzerland), Mineral. Mag., 85, 744–751, 2021.
Biagioni, C., Kasatkin, A., Sejkora, J., Nestola, F., and Škoda, R.: Tennantite-(Cd), Cu6(Cu4Cd2)As4S13, from the Berenguela mining district, Bolivia: the first Cd-member of the tetrahedrite group, Mineral. Mag., 86, 834–840, 2022a.
Biagioni, C., Sejkora, J., Moëlo, Y., Marcoux, E., Mauro, D., and Dolníček, Z.: Tennantite-(Cu), Cu12As4S13, from Layo, Arequipa Department, Peru: a new addition to the tetrahedrite-group minerals, Mineral. Mag., 86, 331–339, 2022b.
Blüml, A., Slačík, J., Straková, S., and Malý, R.: Mineralogical and technological characteristics of the vein S-1 vein at the 7th level of the Radětice deposit, Unpublished report DIAMO SUL, 26 pp., 1984 (in Czech).
Borčinová Radková, A., Jamieson, H., Lalinská-Voleková, B., Majzlan, J., Števko, M., and Chovan, M.: Mineralogical controls on antimony and arsenic mobility during tetrahedrite-tennantite weathering at historic mine sites Špania Dolina-Piesky and L'ubietová-Svätodušná, Slovakia, Am. Miner., 102, 1091–1100, 2017.
Brese, N. E. and O'Keeffe, M.: Bond-valence parameters for solids, Acta Cryst., B47, 192–197, 1991.
Charlat, M. and Lévy, C.: Influence des principales substitutions sur le parametre cristallin de la série tennantite-tétraédrite, Bull. Soc. Franç. Miner. Crist, 98, 152–158, 1975.
Charnock, J. M., Garner, C. D., Pattrick, R. A. D., and Vaughan, D. J.: Coordination sites of metals in tetrahedrite minerals determined by EXAFS, J. Solid St. Chem., 82, 279–289, 1989.
Chetty, R., Bali, A., and Mallik, R. C.: Tetrahedrites as thermoelectric materials: an overview, J. Mater. Chem. C, 3, 12364–12378, 2015.
Criddle, A. J. and Stanley, C. J.: Quantitative data file for ore minerals, third edition, Chapman & Hall, London, ISBN 041246750X, 1993.
Dobbe, R. T. M.: Manganoan-cadmian tetrahedrite from the Tunaberg Cu-Co deposit, Bergslagen, central Sweden, Mineral. Mag., 56, 113–115, 1992.
Flack, H. D.: On enantiomorph-polarity estimation, Acta Cryst., A39, 876–881, 1983.
Garmash, A. A.: Features of the structure of the Zmeinogorsk deposit in Altai – in: Basic questions and methods for studying the structures of ore fields and deposits, Moscow, State scientific and technical publishing house of literature on geology and protection of mineral resources, 566–581, 1960 (in Russian).
Genchi, G., Sinicropi, M. S., Lauria, G., Carocci, A., and Catalano, A.: The effects of cadmium toxicity, Inter. J. Environment. Res., Public Health, 17, 3782, https://doi.org/10.3390/ijerph17113782, 2020.
George, L. L., Cook, N. J., and Ciobanu, C. L.: Minor and trace elements in natural tetrahedrite-tennantite: effects on element partitioning among base metal sulphides, Minerals, 7, 17, https://doi.org/10.3390/min7020017, 2017.
Ghisani, F., Timmo, K., Altosaar, M., Raudoja, J., Mikli, V., Pilvet, M., Kauk-Kuusik, M., and Grossberg, M.: Synthesis and characterization of tetrahedrite Cu10Cd2Sb4S13 monograin material for photovoltaic application, Mat. Sci. Semiconduct. Process., 110, 104973, https://doi.org/10.1016/j.mssp.2020.104973, 2020.
Ghisani, F., Timmo, K., Altosaar, M., Mikli, V., Danilson, M., Grossberg, M., and Kauk-Kuusik, M.: Chemical etching of tetrahedrite Cu10Cd2Sb4S13 monograin powder materials for solar cell applications, Mat. Sci. Semiconduct. Process, 138, 106291, https://doi.org/10.1016/j.mssp.2021.106291, 2022.
Jia, D., Fu, Z., Zhang, H., and Zhao, C.: The first discovery of Cd-freibergite in China, Acta Miner. Sinica, 8, 136–137, 1988 (in Chinese with English abstract).
Johnson, M. L. and Burnham, C. W.: Crystal structure refinement of an arsenic-bearing argentian tetrahedrite, Am. Miner., 70, 165–170, 1985.
Johnson, N. E.: X-ray powder diffraction data for synthetic varieties of tetrahedrite, Powder Diffr., 6, 43–47, 1991.
Johnson, N. E., Craig, J. R., and Rimstidt, J. D.: Compositional trends in tetrahedrite, Can. Mineral., 24, 385–397, 1986.
Johnson, N. E., Craig, J. R., and Rimstidt, J. D.: Effect of substitutions on the cell dimension of tetrahedrite, Cana. Mineral., 25, 237–244, 1987.
Johnson, N. E., Craig, J. R., and Rimstidt, J. D.: Crystal chemistry of tetrahedrite, Am. Miner., 73, 389–397, 1988.
Keim, M. F., Staude, S., Marquardt, K., Bachmann, K., Opitz, J., and Markl, G.: Weathering of Bi-bearing tennantite, Chem. Geol., 499, 1–25, https://doi.org/10.1016/j.chemgeo.2018.07.032, 2018.
Kraus, W. and Nolze, G.: POWDER CELL – a program for the representation and manipulation of crystal structures and calculation of the resulting X-ray powder patterns, J. Appl. Cryst., 29, 301–303, 1996.
Krustok, J., Raadik, T., Kaupmees, R., Ghisani, F., Timmo, K., Altosaar, M., Mikli, V., and Grossberg, M.: Broad-band photoluminescence of donor–acceptor pairs in tetrahedrite Cu10Cd2Sb4S13 microcrystals, J. Phys. D: Appl. Phys., 54, 105102, https://doi.org/10.1088/1361-6463/abce29, 2020.
Kumar, D. S. P., Chetty, R., Rogl, P., Rogl, G., Bauer, E., Malar, P., and Mallik, R. C.: Thermoelectric properties of Cd doped tetrahedrite: Cu12−xCdxSb4S13, Intermetallics, 78, 21–29, 2016.
Levinsky, P., Candolfi, C., Dauscher, A., Tobola, A., Hejtmánek, J., and Lenoir, B.: Thermoelectric propertirs of the tetrahedrite – tennantite solid solutions Cu12Sb4−xAsxS13 and Cu10Co2Sb4−yAsyS13 (0≤x, y≤4), Phys. Chem. Chem. Phys., 21, 4547–4555, 2019.
López Cota, F. A., Díaz-Guillén, J. A., Juan Dura, O., López de la Torre, M. A., Rodríguez-Hernández, J., and Fernández Fuentes, A.: Mechanosynthesis and thermoelectric properties of Fe, Zn, and Cd-Doped P-Type Tetrahedrite: Cu12−xMxSb4S13, Materials, 14, 3448, https://doi.org/10.3390/ma14133448, 2021.
Majzlan, J., Kiefer, S., Herrmann, J., Števko, M., Sejkora, J., Chovan, M., Lánczos, T., Lazarov, M., Gerdes, A., Langenhorst, F., Borčinová Radková, A., Jamieson, H., and Milovský, R.: Synergies in elemental mobility during weathering of tetrahedrite [(Cu,Fe,Zn)12(Sb,As)4S13]: Field observations, electron microscopy, isotopes of Cu, C, O, radiometric dating, and water geochemistry, Chem. Geol., 488, 1–20, https://doi.org/10.1016/j.chemgeo.2018.04.021, 2018.
Makovicky, E. and Karup-Møller, S.: Exploratory studies on substitution of minor elements in synthetic tetrahedrite. Part I. Substitution by Fe, Zn, Co, Ni, Mn, Cr, V and Pb. Unit-cell parameter changes on substitution and the structural role of “Cu2+”, N. Jb. Miner. Abh., 167, 89–123, 1994.
Makovicky, E., Forcher, K., Lottermoser, W., and Amthauer, G.: The role of Fe2+ and Fe3+ in synthetic Fe-substituted tetrahedrite, Mineral. Petrol., 43, 73–81, 1990.
Makovicky, E., Tippelt, G., Forcher, K., Lottermoser, W., Karup-Møller, S., and Amthauer, G.: Mössbauer study of Fe-bearing synthetic tennantite, Can. Mineral., 41, 1125–1134, 2003.
Makovicky, E., Karanović, L., Poleti, D., Balić-Zunić, T., and Paar, W. H.: Crystal structure of copper-rich unsubstituted tennantite, Cu12.5As4S13, Can. Mineral., 43, 679–688, 2005.
Mikuš, T., Vlasáč, J., Majzlan, J., Sejkora, J., Steciuk, G., Plášil, J., Rößler, C., and Matthes, C.: Argentotetrahedrite-(Cd), Ag6(Cu4Cd2)Sb4S13, a new member of the tetrahedrite group from Rudno nad Hronom, Slovakia, Mineral. Mag., 87, 262–270, 2023.
Momma, K., Shimizu, M., Kusaba, Y., and Ohki, Y.: Tetrahedrite-(Mn), IMA 2021-098, in: CNMNC Newsletter 65, Eur. J. Mineral., 34, https://doi.org/10.5194/ejm-34-143-2022, 2022.
Nasonova, D. I., Presniakov, I. A., Sobolev, A. V., Verchenko, V. Y., Tsirlin, A. A., Wei, Z., Dikarev, E. V., and Shevelkov, A. V.: Role of iron in synthetic tetrahedrites revisited, J. Solid St. Chem., 235, 28–35, 2016.
Pattrick, R. A. D.: Microprobe analyses of cadmium-rich tetrahedrites from Tyndrum, Perthshire, Scotland, Mineral. Mag., 42, 286–288, 1978.
Pattrick, R. A. D. and Hall, A. J.: Silver substitution into synthetic zinc, cadmium, and iron tetrahedrites, Mineral. Mag., 47, 441–451, 1983.
Pouchou, J. L. and Pichoir, F.: “PAP” (φρZ) procedure for improved quantitative microanalysis, in: Microbeam Analysis, edited by: Armstrong, J. T., San Francisco Press, San Francisco, 104–106, 1985.
Repstock, A., Voudouris, P., Zeug, M., Melfos, V., Zhai, M., Li, H., Kartal, T., and Matuszczak, J.: Chemical composition and varieties of fahlore-group minerals from Oligocene mineralization in the Rhodope area, Southern Bulgaria and Northern Greece, Mineral. Petrol., 110, 103–123, 2016.
Sejkora, J., Biagioni, C., Vrtiška, L., and Moëlo, Y.: Zvěstovite-(Zn), Ag6(Ag4Zn2)As4S13, a new tetrahedrite-group mineral from Zvěstov, Czech Republic. Mineral. Mag., 85, 716–724, 2021.
Sejkora, J., Biagioni, C., Škácha, P., Musetti, S., and Dolníček, Z.: Hakite-(Cd), IMA 2022-090, in: CNMNC Newsletter 70, Eur. J. Miner., 34, 591–601, 2022.
Sejkora, J., Biagioni, C., Števko, M., Musetti, S., and Peterec, D.: Tetrahedrite-(Cu), Cu12Sb4S13, from Bankov near Košice, Slovak Republic: a new member of the tetrahedrite group minerals, Mineral. Mag., submitted, 2023.
Sheldrick, G. M.: Crystal structure refinement with SHELXL, Acta Cryst., C71, 3–8, 2015.
Škácha, P., Sejkora, J., Palatinus, L., Makovicky, E., Plášil, J., Macek, I., and Goliáš, V.: Hakite from Příbram, Czech Republic: compositional variability, crystal structure and the role in Se mineralization, Mineral. Mag., 80, 1115–1128, 2016.
Škácha, P., Sejkora, J., and Plášil, J.: Selenide mineralization in the Příbram uranium and base-metal district (Czech Republic), Minerals, 7, 91, https://doi.org/10.3390/min7060091, 2017.
Skinner, B. J.: Unit-cell edges of natural and synthetic sphalerites, Am. Miner., 46, 1399–1411, 1961.
Štekl, J., Sedláček, J., Pech, V., Müller, L., and Měrák, J.: Radětice shaft – vertical development of the S-1 vein, unpublished report DIAMO SUL, 60 pp., 1981 (in Czech).
Štekl, J., Chyba, F., Pech, V., and Müller, L.: Final report of project VP – shaft Radětice – vein S-1 – 3rd level, unpublished report DIAMO SUL, 36 pp., 1984a (in Czech).
Štekl, J., Chyba, F., Pech, V., and Müller, L.: Final report of project VP – shaft Radětice – vein S-1 – 7th level, unpublished report DIAMO SUL, 36 pp., 1984b (in Czech).
Suekuni, K., Tomizawa, Y., Ozaki, T., and Koyano, M.: Systematic study of electronic and magnetic properties for Cu12−xTMxSb4S13 (TM = Mn, Fe, Co, Ni, and Zn) tetrahedrite, J. Appl. Phys., 115, 143702, https://doi.org/10.1063/1.4871265, 2014.
Timofeevskiy, B. A.: On the ore content and stratigraphy of the Zmeinogorsk deposit, Soviet Geology, 2–3, 17–29, 1940 (in Russian).
Voropaev, A. V., Spiridonov, E. M., and Shchibrik, V. I.: Tetrahedrite-Cd – first find in USSR, Dokl. Akad. Nauk SSSR, 300, 1446–1448, 1988 (in Russian).
Voudouris, P. C., Spry, P. G., Sakellaris, G. A., and Mavrogonatos, C.: A cervelleite-like mineral and other Ag-Cu-Te-S minerals [Ag2CuTeS and (Ag,Cu)2TeS] in gold-bearing veins in metamorphic rocks of the Cycladic Blueschist Unit, Kallianou, Evia Island, Greece, Mineral. Petrol., 101, 169–183, 2011.
Wang, Y., Chen, R., Gu, X., Nestola, F., Hou, Z., Yang, Z., Dong, G., Hu, G. and Qu, K.: Tetrahedrite-(Ni), Cu6(Cu4Ni2)Sb4S13, the first nickel member of tetrahedrite group mineral from Luobusa chromite deposit, Tibet, China, Am. Miner., 108, 1984–1992, https://doi.org/10.2138/am-2022-8761, 2023.
Warr, L. N.: IMA-CNMNC approved mineral symbols, Mineral. Mag., 85, 291–320, 2021.
Wedepohl, K. H.: The composition of the continental crust, Geochim. Cosmochim. Ac., 59, 1217–1232, 1995.
Welch, M. D., Stanley, C. J., Spratt, J., and Mills, S. J.: Rozhdestvenskayaite Ag10Zn2Sb4S13 and argentotetrahedrite Ag6Cu4(Fe2+,Zn)2Sb4S13: two Ag-dominant members of the tetrahedrite group, Eur. J. Miner., 30, 1163–1172, https://doi.org/10.1127/ejm/2018/0030-2773, 2018.
Weller, D. P. and Morelli, D. T.: Tetrahedrite thermoelectrics: from fundamental science to facile synthesis, Front. Electron. Mater., 2, 913280, https://doi.org/10.3389/femat.2022.913280, 2022.
Wilson, A. J. C. (Ed.): International Tables for Crystallography Volume C: Mathematical, Physical and Chemical Tables, Kluwer Academic Publishers, Dordrecht, the Netherlands, 1992.
Wuensch, B. J.: The crystal structure of tetrahedrite, Cu12Sb4S13, Z. Kristallogr., 119, 437–453, 1964.
Wuensch, B. J., Takéuchi, Y., and Nowacki, W.: Refinement of the crystal structure of binnite, Cu12As4S13, Z. Kristallogr., 123, 1–20, https://doi.org/10.1524/zkri.1966.123.16.1, 1966.
Xu, Y. N. and Ching, W. Y.: Electronic, optical, and structural properties of some wurtzite crystals, Phys. Rev., B48, 4335–4351, 1993.