the Creative Commons Attribution 4.0 License.
the Creative Commons Attribution 4.0 License.
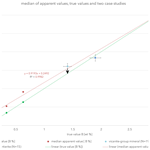
Appearance, study and a possible correction for boron: a phenomenon in ultra-soft X-ray measurements using a synthetic multilayer crystal and the EPMA
Franziska Daniela Helena Wilke
When measuring boron (B) in tourmalines calibrated with schorl, no deviations in the peak intensities could be detected with a proven analysis protocol and using the Mo/B4C multilayer crystal LDEB. It is only when boron is detected in natural and experimental samples, some with significantly lower boron concentrations than in tourmalines, that irregularities in the analysis become visible. This phenomenon is known but has not been analytically investigated so far. Using four natural and artificial solids with boron concentrations from 0.035 wt %–3.14 wt % B, an apparent linear trend line was drawn. The intersect of that trend line with the y axis represents the detection limit of boron, which is of about 0.25 wt % B. The discrepancy between the apparent and the true value trend lines at boron concentrations of 0.25 wt %–2.1 wt % B shows that a correction is necessary. At higher boron concentrations, the discrepancy between the apparent and true value trend lines is within the uncertainty of electron microprobe analysis (EPMA) and disappears completely up to boron concentrations of about 3 wt %.
- Article
(1842 KB) -
Supplement
(380 KB) - BibTeX
- EndNote
Boron (B) is a light lithophile element with the atomic number 5 whose X-ray Kα emission is observed in the field of 0.183 keV at 5 kV or higher. Together with lithium, boron (and its isotopes) gained attention for its role in hydrothermal fluids to track the circulation of matter in general and specifically of light elements down to the deep mantle. In quite a lot of nominally boron-free phases, boron is detected in small quantities of some hundreds or thousands of parts per million (ppm). Apart from well-known tourmaline, kornerupine or dumortierite, also dense hydrous magnesium silicates like the 10 Å-phase from the deep mantle or coesite from the deep crust to upper mantle can contain B. In HP and UHP phases boron and hydrogen can together substitute Si, meaning that tetrahedral boron, as well as planar coordinated boron, is possible (e.g., Kutzschbach, 2017).
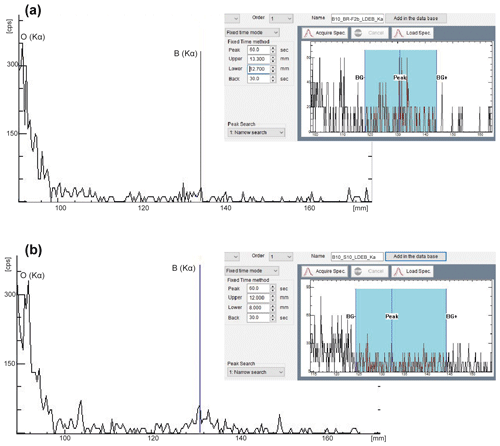
Figure 1Peak position of boron using an LDEB diffraction crystal and determination of the background (± BG). Panel (a) refers to the standard glass BR-F2 and (b) to the Harvard-Schorl S10. With the wide qualitative scan, one can best examine the width of the peaks under consideration and neighboring peaks, while in the narrow scan (here the inserts) the background can best be determined (limits of the blue area).
Boron can be chemically separated from its silicate matrix in minerals and rocks by alkaline fusion with the advantage of simplicity, low degree of loss and fractionation of boron isotopes (e.g., Owens et al., 1982; Kasemann et al., 2001). The boron concentration and its isotopes can be determined thereafter by inductively coupled plasma mass spectrometry (ICP-MS) down to 20 ppm or thermal ionization mass spectrometry (TIMS). In situ, boron can be analyzed by secondary ion mass spectrometry (SIMS; e.g., in Rosner et al., 2008) and by electron microprobe analysis (EPMA).
Table 1Major constitutes and boron of used standards (denoted by a) and test materials. b signifies an informative value.
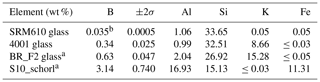
Measuring light elements from Be (Z=4) up to F (Z=9) using EPMA requires synthetic multilayer crystals with a spacing of >10 nm to diffract very soft X-rays. Since the acquisition of the JEOL 8500F microprobe with a thermal field emission cathode in 2007 at the GFZ in Potsdam, we have been working with synthetic multilayer crystals. In addition to the detection of light elements, low-energy Lα lines of, for example, Fe and Cu at low voltages, for example, 5 kV can be identified. In 2020, a new JEOL 8530F+ was installed, and the previously used multilayer crystal LDEB was reinstalled in it.
During measurements of boron in tourmalines calibrated with schorl and elbaite standards from the Harvard Mineralogical Museum (e.g., Wiedenbeck et al., 2021, and references therein), deviations in the peak intensities could not be detected by using the Mo/B4C multilayer crystal LDEB. A well-designed analytical protocol (e.g., as in Cheng et al., 2019), the same matrix, and comparably high boron concentrations in the standard and the unknown sample prevented unpleasant phenomena. Only when detecting boron with, in part, considerably lower concentrations in natural and experimental phases did irregularities become visible in the analysis. This was for the first time experienced in synthetic diamond that was contaminated with boron to achieve a blue color. Subsequent studies have shown that a boron peak can be detected in samples that are presumed and proven to contain no boron when a Mo/B4C multilayer crystal is used. This phenomenon is known and was explained as some of the incident X-rays induce X-ray fluorescence of the boron atoms in the B.1C spacer layer of the multilayer crystal (Kobayashi et al., 1995). This leads to the fact that supposedly more boron is detected in the minerals than is actually present and that the detection limit could not be as low as for other elements. Using B-bearing and B-free minerals and certified glasses with different but markedly lower boron concentrations than tourmaline, a correction curve was created and tested and is presented herein.
Most of the boron X-rays that escape from the diffracting crystal and are detected show up as a smooth increase in background intensity and could be avoided by setting the background accordingly. Using JEOL software, it is best to qualitatively scan a material with a high boron content to know the peak position of boron for sure. Then, for each unknown boron-containing phase, the same peak scan should be performed on the material. The determined boron peak can then be filtered out. By means of the adjustable limits for the negative and positive background, this limit can be set at a position that results in the smallest possible intensity deduction and thus loss of intensity (Fig. 1).
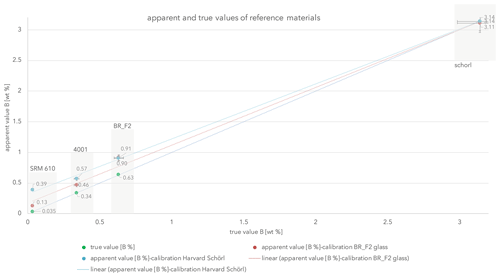
Figure 2Analytical results of our reference materials (in swaths). Error bars symbolize 5 % of uncertainty.
Unfortunately, a small portion of the induced boron Kα X-rays is probably scattered by the molybdenum reflective layer. Some of these X-rays in turn constructively interfere with the 2θ diffraction angles for boron, resulting in a boron peak.
Beside the Harvard schorl, three certified glasses with a considerably lower boron content than tourmaline were used for calibration and test measurements (Table 1). Since mineral phases with a certified boron content are very rare, it was necessary to resort to glasses.
The Harvard standard tourmaline schorl (Wiedenbeck et al., 2021; Dyar et al., 2001) was used for calibration and test measurements. Further, BR F2 was used for calibration and testing and is a glass manufactured by Breitländer Eichproben und Labormaterial GmbH, Hamm.
Glass 4001 was used for testing only and is a Czech certified reference material from the Metrological Institute in Brno. From the National Institute of Standards and Technology (NIST) the SRM610 glass was used to yield the boron “bottom line”. Therein, boron is not certified but acknowledged with 351 ppm (0.0351 wt %) after isotope dilution mass spectrometry (IDMS) which has a max uncertainty of 1.4 % (Vogl, 2012). In Table 1 one can find the certified boron values with 2σ uncertainties and the four major elemental constituents of all standard materials.
All standard and test materials were previously coated with 20 nm carbon in the same coater (data provided by the maintenance company) using carbon filaments from one roll and under the same vacuum and current conditions. Using these conditions, the thickness of the carbon coat remains the same and is monitored once in a while during maintenance of the coater. This technical aspect is important because different coating thicknesses would have an impact on the low-energy X-rays of the light element boron. Simultaneous sputtering of all objects was not possible due to space constraints. The measurements at the EPMA were repeated within several days to avoid or detect technical inaccuracies due to either my measurement technique or the hardware. A voltage of 10 kV and a current of 20 nA using 10 µm probe size was applied. The LDEB diffracting crystal with a 2d spacing of ca. 14.5 nm was used. This crystal has a guaranteed peak background (P B) ratio of 120. Its background is higher than that of an LDE2 or STE crystal, but the peak intensity is with 8.00×105 cps µA−1 a minimum of 5 times better than both other crystals (all manufacturer data). There are no peak interferences with elements present in the used phases, e.g., with oxygen (Fig. 1). The accompanied measurement gas is P10 (Ar 90 %, CH4 10 %). Data reduction was done with the a φ(ρZ) correction scheme (CITZAF; Armstrong, 1995). Further details are given in Table 2.
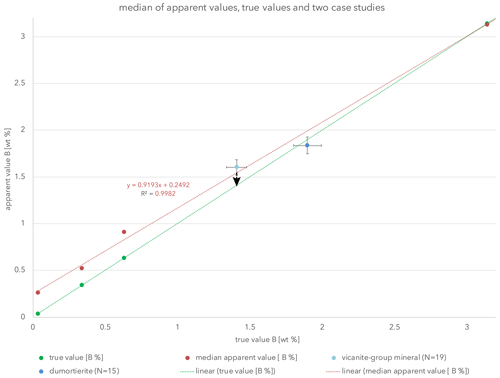
Figure 3Linear trend lines (red) for the apparent values and (green) for the true values. The red line crosses the y axis at 0.249, which should be seen as the DL for boron on LDEB. The arrow at the vicanite group mineral data point indicates the correction of B.
Using the above-mentioned three referenced glasses and the referenced Harvard schorl, results given in Table 3 were achieved. Using these results linear apparent value trend lines were drawn (Fig. 2), resembling the obtained results with an R2 value better than 0.995. Those apparent values forming a line symbolize the “credit side”.
The true value linear trend line (R2=1) can be interpreted as the debit line. Since the boron value from SRM610 (350 ppm) is not certified, one needs to interpret it with caution.
In Fig. 3 the true value trend line as in Fig. 2 was drawn, but the two apparent values of each test material were combined and a median apparent trend line drawn. With an R2 value of 0.998, the precision of the eight yielded values are well represented.
The major outcome of this study is that the discrepancy between the apparent and true values diminishes at 2.8 wt % boron and is already within uncertainty at 2.1 wt %. Taking an analytical uncertainty of ca. 5 % for values of 1 wt %–3 wt % boron into account, corrections are therefore appropriate for concentrations of less than 2.1 wt % boron.
The intercept of the apparent value trend line in Fig. 3 with the y axis is at 0.249, meaning that the absolute detection limit (DL) of boron measurements using the LDEB crystal should be seen at ca. 0.25 wt % (2500 ppm).
The results presented here could be very valuable for EPMA users investigating low boron concentrations in minerals. Nevertheless, a critical consideration of all the results obtained is necessary. From the above-mentioned small fraction of the induced boron X-rays scattered by the molybdenum reflective layer, apparently only a part of it constructively interferes with the diffraction angles for boron, resulting in a boron peak. This means that the occurrence of this phenomenon can be nonlinear. This can be seen in the following examples.
During the course of two independent studies, the herein-presented findings were tested. The Dora-Maira natural dumortierites, studied by, for example, Ferraris et al. (1995) and Chopin et al. (1995) and recently compared to experimentally formed HP dumortierite by Wunder et al. (2018), were analyzed using the same conditions as previously described (10 kV, 20 nA, 10 µm, boron calibrated with schorl on LDEB). The dumortierites from two different localities showed nearly identical boron concentrations giving an average (N=15) of 1.84 wt % B. If this result is presented graphically and an error of 5 % is applied, this value fits to the true value trend line and does not need a correction. Using the chi2 statistic test to quantify the shift or the fitting to the true value trend line, the calculated value is 0.998. The table of the chi2 distribution shows that with the calculated value for each degree of freedom and a significance level of 5 %, the calculated chi2 value is smaller. Hence, there is no significant difference in apparent and true value.
For the analysis of a mineral of the vicanite group, adjustments of the analytical protocol towards 20 kV, 40 nA and 30 µm probe size were necessary because rare earth elements (REEs), U, Th and Pb were present. Calibration was performed on BR_F2 as a low boron concentration was expected. Boron was calibrated on BR_F2 under the same conditions as the measurements were performed. An average value (N=19) of 1.62 wt % B was determined. Based on the present diagram, the boron values were corrected graphically to an average of 1.4 wt % B, which agrees with the structural data obtained later. The manuscript for this is in preparation, so no further details can be given.
An extrapolation and extension of the herein-presented apparent and true value trend lines towards boron concentrations of reedmergnerite, danburite and boracite are in preparation. The expectation is that a calibration using tourmaline with 3.14 wt % B will probably not be appropriate. Therefore, establishing a new calibration material is under way as well. Further, a model fit might be available by then to enable boron correction across the entire concentration range.
All data derived and used in and for this research are presented in the enclosed tables and figures or in Supplement Tables S1 and S2. The latter presents the apparent and respective true boron values as extracted from the presented graph (Fig. 3).
The supplement related to this article is available online at: https://doi.org/10.5194/ejm-35-59-2023-supplement.
FDHW did all preparatory work, the measurements, and the data evaluation and interpretation. FDHW created all tables and drew all figures herself. Therefore, the right to distribute her figures, tables and text lies with FDHW.
The author has declared that there are no competing interests.
Publisher’s note: Copernicus Publications remains neutral with regard to jurisdictional claims in published maps and institutional affiliations.
I would very much like to thank Oona Appelt and Bernd Wunder, both from GFZ, for very helpful discussions. Moreover, working times at the EPMA together with Jürgen Börder were highly appreciated. He also supported me with additional references. Nicolas Rividi, another anonymous reviewer and the associate editor Vincent van Hinsberg are thanked for their valuable comments on the manuscript. Financial support was provided by the internal GFZ call “The things we do when we can't do the things we do” during the Corona pandemic.
The article processing charges for this open-access publication were covered by the Helmholtz Centre Potsdam – GFZ German Research Centre for Geosciences.
This paper was edited by Vincent van Hinsberg and reviewed by Nicolas Rividi and one anonymous referee.
Armstrong, J. T.: CITZAF: a package of correction programs for the quantitative Electron Microbeam X-Ray-Analysis of thick polished materials, thin-films, and particles, Microbeam. Anal., 4, 177–200, 1995.
Cheng, L., Zhang, C., Li, X., Almeev, R. R., Yang, X., and Holtz, F.: Improvement of electron probe microanalysis of boron concentration in silicate glasses, Microsc. Microanal., 25, 874–882, 2019.
Chopin, C., Ferraris, G., Ivaldi, G., Schertl, H. P., Schreyer, W., Compagnoni, R., Davidson, C., and Davis, A. M.: Magnesiodumortierite, a new mineral from very-high-pressure rocks (Western Alps), Part II: Crystal chemistry and petrological significance, Eur. J. Mineral., 7, 525–535, 1995.
Dyar, M. D., Wiedenbeck, M., Robertson, D., Cross, L. R., Delaney, J. S., Ferguson, K., and Yates, M.: Reference minerals for the microanalysis of light elements, Geostandard Newslett., 25, 441–463, 2001.
Ferraris, G., Ivaldi, G., and Chopin, C.: Magnesiodumortierite, a new mineral from very-high-pressure rocks (Western Alps), Part I: Crystal structure, Eur. J. Mineral., 7, 167–174, 1995.
Kasemann, S., Meixner, A., Rocholl, A., Vennemann, T., Rosner, M., Schmitt, A. K., and Wiedenbeck, M.: Boron and oxygen isotope composition of certified reference materials NIST SRM 610/612 and reference materials JB-2 and JR-2, Geostandard Newslett., 25, 405–416, 2001.
Kutzschbach, M.: The effect of tetrahedral boron in tourmaline on the boron isotope fractionation between tourmaline and fluid: improving tourmaline as a petrogenetic indicator, doctor thesis, Technische Universität Berlin, 135 pp., https://doi.org/10.14279/depositonce-5893, 2017.
Kobayashi, H., Toda, K., Kohno, H., Arai, T., and Wilson, R.: The study of some peculiar phenomena in ultra-soft X-ray measurements using synthetic multilayer crystals, Adv. X-Ray Anal., 38, 307–312, 1994.
Owens, J. W., Gladney, E. S., and Knab, D.: Determination of boron in geological materials by inductively-coupled plasma emission spectrometry, Anal. Chim. Acta, 135, 169–172, 1982.
Rosner, M., Wiedenbeck, M., and Ludwig, T.: Composition induced variationsin SIMS instrumental mass fractionation during boron isotope ratio measurements of silicate glass, Geostandard Geoanal. Res., 32, 27–38, 2008.
Vogl, J.: Measurement uncertainty in single, double and triple isotope dilution mass spectrometry, Rapid Commun. Mass. Sp., 26, 275–281, 2012.
Wiedenbeck, M., Trumbull, R. B., Rosner, M., Boyce, A., Fournelle, J. H., Franchi, I. A., and Wilke, F. D. H.: Tourmaline reference materials for the in situ analysis of oxygen and lithium isotope ratio compositions, Geostandard Geoanal. Res., 45, 97–119, 2021.
Wunder, B., Kutzschbach, M., Hosse, L., Wilke, F. D., Schertl, H. P., and Chopin, C.: Synthetic [4] B-bearing dumortierite and natural [4] B-free magnesiodumortierite from the Dora-Maira Massif: differences in boron coordination in response to ultrahigh pressure, Eur. J. Mineral., 30, 471–483, 2018.