the Creative Commons Attribution 4.0 License.
the Creative Commons Attribution 4.0 License.
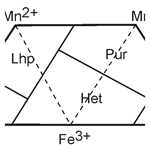
Nomenclature of the triphylite group of minerals
Lyudmila M. Lyalina
Ekaterina A. Selivanova
Frédéric Hatert
In this paper we present a Commission on New Minerals, Nomenclature and Classification of the International Mineralogical Association (IMA-CNMNC)-accepted scheme for the classification and nomenclature of the triphylite group of minerals. The general formula of those minerals is M1M2TO4, where M1 and M2 refer to cations in an octahedral coordination: M1 = □, Na, Li; M2 = Mn2+, Fe2+, Mg, Fe3+, Mn3+, and T to tetrahedrally coordinated P5+ cations. The group contains the Li-bearing phosphates triphylite [LiFe2+(PO4)] and lithiophilite [LiMn2+(PO4)] and their oxidation products heterosite [Fe3+(PO4)] and purpurite [Mn3+(PO4)], as well as the Na-bearing phosphates natrophilite [NaMn2+(PO4)] and karenwebberite [NaFe2+(PO4)]. The Li–Mg-bearing phosphate simferite has been redefined as LiMg(PO4). Ferrisicklerite and sicklerite correspond to intermediate phases in the triphylite–heterosite and lithiophilite–purpurite solid solutions; consequently, according to the CNMNC dominant-constituent rule, they are discredited. A new mineral oxidation sequence is defined, which considers the different oxidation capacity of iron and manganese, and therefore replaces the traditional Quensel–Mason sequence. The formula calculation procedure for Li-bearing species, based on electron microprobe analyses and single-crystal X-ray diffraction data, is also described.
- Article
(1390 KB) - Full-text XML
-
Supplement
(112 KB) - BibTeX
- EndNote
The triphylite group includes seven phosphate mineral species, characterized by the well-known olivine-type crystal structure (Brush et al., 1890; Alberti, 1976; Fontan et al., 1976; Hatert, 2012; Hatert et al., 2012; and others). Their general formula is M1M2TO4, where M1 and M2 refer to cations in an octahedral coordination (M1 = □, Na, Li; M2 = Mn2+, Fe2+, Fe3+, Mn3+, Mg), and T corresponds to the tetrahedrally coordinated P5+ cation (Losey et al., 2004).
Triphylite, heterosite, sicklerite, ferrisicklerite, lithiophilite, and purpurite are common Li-bearing minerals found in granitic pegmatites. They are connected to each other by heterovalent substitutions following the mechanism Li+ + (Fe2+,Mn2+) ↔ □ + (Fe3+,Mn3+), leading to the so-called Quensel–Mason oxidation sequence (Schmid-Beurmann et al., 2013; Baijot et al., 2012; Hatert, 2012; Hatert et al., 2012). These phosphates were initially only of mineralogical interest; however, electrochemical studies carried out in the 1990s (Padhi et al., 1997) caused an avalanche-like increase in the number of publications devoted to these compounds, both of natural and synthetic origin. Nowadays, triphylite-like phosphates are considered one of the most effective cathode material for lithium-ion batteries (Fehr et al., 2007; Hatert, 2012; Yakubovich et al., 2020, and references therein).
Sodium-bearing phosphates, natrophilite [NaMn2+(PO4)] (Brush et al., 1890) and the relatively recently approved karenwebberite [NaFe2+(PO4)] (Vignola et al., 2013), are structurally identical to olivine-type Li–(Fe,Mn) phosphates and consequently belong to the triphylite group of minerals, too. Simferite, Li(Mg,Fe3+,Mn3+)2(PO4)2, with an olivine-type crystal structure and half-occupied M1 site, is also structurally related to triphylite-type phosphates (Yakubovich et al., 1989).
Ferrisicklerite and sicklerite appear to be intermediate phases of the oxidation of triphylite–lithiophilite into heterosite–purpurite, where ferrous ion is totally or partially oxidized into ferric iron: Li(Fe2+,Mn2+)(PO4) triphylite–lithiophilite → Li1−xMnFe(PO4) sicklerite–ferrisicklerite → (Fe3+,Mn3+)(PO4) heterosite–purpurite. In some cases, it may be difficult to identify these minerals from their chemical analyses due to the gradual transition from one mineral to another during the oxidation process, difficulties in pure sampling for analysis, and limited analytical capabilities (for example, definition of trivalent manganese is not a routine procedure). Consequently, optical properties are often used to confirm the identification, and most authors consider that lithiophilite and triphylite are colorless, while sicklerite is deep orange, and ferrisicklerite is reddish-brown chocolate or brownish black in the thin section (Fransolet et al., 1986; Hatert et al., 2012; Baijot et al., 2012; Roda-Robles et al., 2014). The local analytical methods only partly solve the situation for these minerals, since the electron probe microanalysis (EPMA) – the most commonly used local method for determining a chemical composition – does not allow us to determine the lithium content and distinguish the valence states of iron and manganese. A direct lithium measurement by the laser ablation inductively coupled plasma mass spectrometry (LA-ICP-MS) method shows a big margin of error (Svetov et al., 2015), while secondary ion mass spectrometry (SIMS) techniques are much more reliable for determining lithium. The higher standard deviations generally pertain to the low or very low Li concentrations (Hatert et al., 2011a). Therefore, researchers continue either to use indirect criteria for mineral definition or to apply different analytical method combinations, which are leading to a more or less acceptable result.
In this paper we present a CNMNC-approved nomenclature scheme for the triphylite group, in which end-member mineral formulae are defined (Miyawaki et al., 2022). Simferite is redefined as LiMgPO4, and ferrisicklerite and sicklerite are discredited, since they constitute intermediate members in solid solutions. We also discuss the interpretation of chemical analyses in these complex minerals affected by variable valence states during their oxidation processes.
Minerals of the triphylite group (Table 1) crystallize in the space group Pbnm (Finger and Rapp, 1969; Eventoff et al., 1972; Moore, 1972; Alberti, 1976; Losey et al., 2004; Fehr et al., 2007; Hatert et al., 2012; Vignola et al., 2013; Schmid-Beurmann et al., 2013) and show the olivine crystal structure characterized by two chains of edge-sharing octahedra parallel to the c axis. The first chain is constituted by the M1 octahedra occupied by Li, Na, or vacancies, while the second chain is formed by the M2 sites occupied by Fe, Mn, and Mg (Fig. 1). The occupancy of the M1 position varies from 0 to 1 in Li-bearing species, and it approaches 1 in Na-bearing species. The structural formula of triphylite-type phosphates is M1M2TO4, where T corresponds to P5+ in tetrahedral coordination (Losey et al., 2004). The cation ordering between M1 and M2, first defined for natrophilite (Moore, 1972), is the main crystal–chemical difference between triphylite-type phosphates and silicates of the forsterite–fayalite series, in which Fe and Mg are disordered over the two sites.
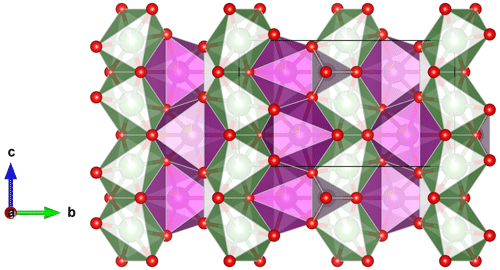
Figure 1The crystal structure of lithiophilite, projected perpendicular to the a axis (Hatert et al., 2012). The M1 octahedra containing Li are green, the M2 octahedra containing Fe and Mn are purple, and the PO4 tetrahedra are light grey.
Table 1Chemical composition, cell dimensions (space group Pbnm, Z=4), and optical data for triphylite-group minerals. RI stands for refractive index.
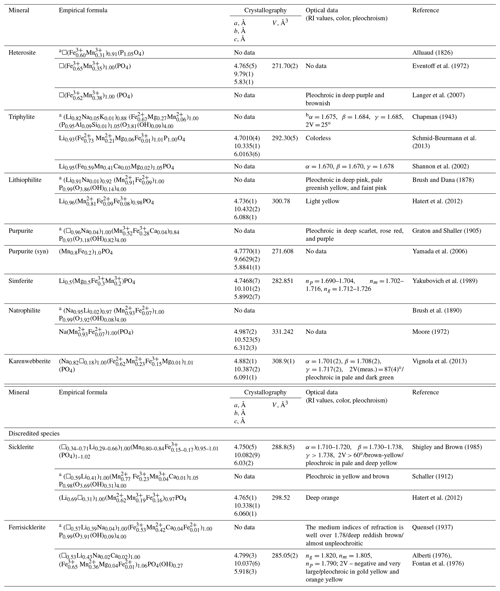
a Calculated on the basis of O = 4. b “The indices of refraction and optic angle were determined for sodium light” (Chapman, 1943).
Oxidation of Fe2+ to Fe3+ and of Mn2+ to Mn3+ produces Li vacancies at the M1 sites, which are necessary to maintain charge balance. The alignment of M1 octahedra along the c axis explains why the [001] direction becomes an easy and fast pathway for Li/Na leaching during oxidation or absorption when conditions lead to the reduction in Fe and Mn. This is the principle widely in used Li-ion batteries based on olivine-type phosphates (Rakovan, 2005; Yakubovich et al., 2020).
The main chemical, crystallographic, and optical data for triphylite-group minerals are summarized in Table 1. These data are those of the first-described occurrence of the species, as well as supplementary references for which complete structural and/or chemical datasets were available. Heterosite is the oldest mineral of the group (Alluaud, 1826), but in the present case, the name of the more common species triphylite (Fuchs, 1834) was given for the group, in contradiction with the precedence rule (Mills et al., 2009). Heterosite was followed by the discoveries of triphylite LiFe2+(PO4) (Fuchs, 1834), lithiophilite LiMn2+(PO4) (Brush and Dana, 1878), and purpurite □Mn3+(PO4) – another completely leached and oxidized phase (Graton and Schaller, 1905) – and then by the intermediate products, sicklerite (Schaller, 1912), and ferrisicklerite (Quensel, 1937).
As mentioned above, the accurate determination of minerals in the Quensel–Mason sequence is delicate due to the difficulties in the determination of Fe and Mn valence states and of the lithium contents. Methods based on unit-cell parameter variations do not solve the problem because these parameters are affected by several variables simultaneously, e.g., the ratio; the presence of Mg, Fe3+, and other impurities (Na, Ca, etc.); and the sample homogeneity (Fransolet et al., 1984) (Table 1). Moreover, for natural purpurite, crystallographic data are absent, since the sample considered purpurite by Björling and Westgren (1938) indeed corresponds to heterosite (Table 1).
Triphylite, heterosite, sicklerite, ferrisicklerite, lithiophilite, and purpurite are the most common and well-studied members of the group, and their investigations aimed to elucidate the formation conditions and the mechanism of topotactic reactions leading to this suite of phosphate minerals and therefore contributed to the reconstruction of the physico-chemical conditions of phosphate mineral formation in granitic pegmatite. The other phosphates of the group are very rare minerals, which are described below.
Simferite shows the olivine-type crystal structure, with the M1 site being half occupied by Li and the M2 site dominantly occupied by Mg (Yakubovich et al., 1989). Magnesium-rich triphylite samples were also described from a quartz–albite pegmatite at Newport, New Hampshire (Chapman, 1943) (Table 1). Natrophilite is almost as old as lithiophilite (Brush et al., 1890), but it is still an extremely rare mineral. There is a reason to assume that natrophilite is a product of the Na-metasomatic exchange reaction with lithiophilite so that natrophilite may occur only in Mn-rich geological environments. Natrophilite was the first olivine-type phosphate with a confirmed ordered cationic distribution over the two M sites (Moore, 1972). Finally, karenwebberite can be regarded as the Fe equivalent of natrophilite or as the Na equivalent of triphylite (Table 1). In progressive oxidation conditions, karenwebberite is affected by the substitution mechanism Na+ + Fe2+ → □ + Fe3+, leading to heterosite.
In Fleischer's Glossary of Mineral Species (2008), in addition to the minerals listed in Table 1, marićite (NaFePO4; a=6.861(1), b=8.987(1), c=5.045(1), Pmnb) and buchwaldite (NaCaPO4; a=5.17(3), b=9.26(5), c=6.74(3), Pmn21) are also included in the triphylite group, since they show the same general formula M1M2PO4. However, these minerals have a topology distinct from that of olivine (Struman et al., 1977; Le Page and Donnay, 1977). Marićite is also the high-temperature dimorph of karenwebberite, and the transition between the two polymorphs of NaFe2+(PO4) occurs around 325 ∘C (P = 100 bars) (Vignola et al., 2013). A similar high-temperature synthetic polymorph with a marićite-like structure is known for natrophilite (Hatert et al., 2006, 2011a) and can be obtained by heating a synthetic analog of natrophilite (Jana et al., 2020).
The formulae of the nine species included in the triphylite group are given in Table 2, with the formulae occurring in the IMA-CNMNC before this nomenclature revision (December 2022), and the new end-member formula is also given.
Examination of the mineral formulae previously occurring in the CNMNC list shows several inconsistencies:
-
The same formula is reported for sicklerite and lithiophilite – LiMn2+(PO4).
-
The formulae of ferrisicklerite, Li1−x(Fe3+,Mn2+)(PO4), and of simferite, Li(Mg,Fe3+,Mn3+)2(PO4)2, are not end-member formulae.
-
The formula of simferite is expressed on the basis of two (PO4) groups per formula unit, while the formulae of other members of the group are expressed on the basis of one (PO4) group per formula unit.
-
Dual names are sometimes used in the literature for some of these minerals, i.e., “lithiophilite–sicklerite” (Hatert et al., 2011b).
-
Major uncertainties exist in mineral identification. This is explained, for example, by Roda-Robles et al. (2014): “a wide range of Li content, which could be related to different degrees of leaching of Li from the primary triphylite, giving intermediate values between triphylite and ferrisicklerite”. These difficulties are caused by the contradictions between the traditional definition of ferrisicklerite and sicklerite as separate species (IMA List of Minerals, December 2022) or series (Fontan et al., 1976) containing a comparable content of divalent and trivalent components in the M2 site and the IMA rules for mineral species determination based on crystal–chemical principles. It is noteworthy that the problem of nomenclature of olivine-type phosphates in general and the question of distinction between lithiophilite and sicklerite, in particular, have already been raised (Hatert et al., 2012).
We believe that these mineral species should be determined only on the basis of their fundamental characteristics, namely their chemical composition and crystal structure, and that they should be characterized by unique end-member formulae. For that reason, we submitted a proposal to the CNMNC, which was accepted in October 2022 (Miyawaki et al., 2022).
The heterovalent substitution mechanism Li+ + (Fe2+,Mn2+) ↔ □ + (Fe3+,Mn3+) connects the minerals in the triphylite–heterosite (Fe mineral species) and in the lithiophilite–purpurite series (Mn mineral species). Moreover, homovalent substitutions Fe2+ ↔ Mn2+ in the triphylite–lithiophilite series and Fe3+ ↔ Mn3+ in the heterosite–purpurite series are also present. Consequently, the four cations Fe2+, Mn2+, Fe3+, and Mn3+ occur in the M2 site of these phosphates.
Due to the difference in oxidation potential of iron and manganese (Schmid-Beurmann et al., 2013), we can assume that the manganese oxidation will start after the complete transformation of Fe2+ into Fe3+. As a consequence, in the M2 site, only the following cation combinations are possible: (1) Fe2+, Mn2+; (2) Fe2+, Mn2+, Fe3+; (3) Mn2+, Fe3+; (4) Mn2+, Fe3+, Mn3+; and (5) Fe3+, Mn3+. Ternary diagrams Fe2+–Mn2+–Fe3+ and Mn2+–Fe3+–Mn3+ can therefore be used to represent the isomorphic substitutions in the M2 site (Fig. 2a). Triangles show a common Mn2+–Fe3+ edge and can be combined along this line (Fig. 2b, c).
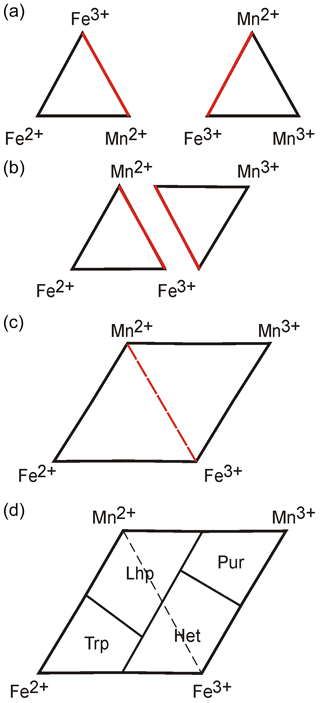
Figure 2Diagrams for the representation of chemical compositions of (Fe,Mn)-olivine-type phosphates. Abbreviations: Trp – triphylite, Lhp – lithiophilite, Pur – purpurite, and Het – heterosite.
The “dominant-valency rule” for defining minerals in solid solution series with heterovalent substitutions can be applied here (Fig. 3b in Hatert and Burke, 2008). Boundaries between mineral species should ideally be defined as shown in Fig. 2d. In this case, we do not consider “ferrisicklerite” and “sicklerite” as valid mineral species, since they were defined on the basis of their optical features: both of them appear as brownish phases forming between triphylite and heterosite and between lithiophilite and purpurite, respectively. Since these phases are intermediate members of solid solutions, it is impossible to establish valid end-member formulae for them, and, consequently, they cannot be maintained as valid mineral species. We have consequently suggested discrediting both ferrisicklerite and sicklerite; this discreditation was approved by the IMA-CNMNC (Miyawaki et al., 2022).
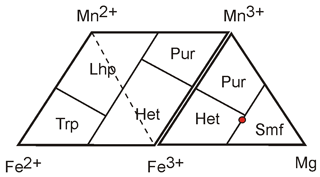
Figure 3Diagram for the representation of chemical compositions of Mn, Fe, and Mg mineral species. Abbreviations: Trp – triphylite, Lhp – lithiophilite, Pur – purpurite, Het – heterosite, and Smf – simferite.
Triphylite, lithiophilite, heterosite, and purpurite are the end-members of solid solution series, and their compositions correspond to the Fe2+, Mn2+, Fe3+, and Mn3+ vertices of the diagrams, respectively. In the case of magnesium-bearing species, the diagram can be completed by the Fe3+–Mn3+–Mg triangle (Fig. 3). According to Yakubovich et al. (1989), the composition of simferite is Li(MgFeMn)2(PO4)2, which is graphically represented by the point exactly between heterosite and the hypothetical end-member LiMg(PO4) (Fig. 3). This composition, however, was obtained from crystal structural data and cannot be considered the empirical chemical composition of the species.
Bayrakov et al. (2005) re-analyzed simferite samples by electron microprobe, obtaining two analyses in which they considered the theoretical content of 1 Li pfu, leading to 5.45 wt. % and 5.35 wt. % Li2O. They also measured lithium independently by an unknown technique, leading to 6.12 wt. % Li2O (Ercit et al., 2006). By using this measured lithium content, we can calculate the two following empirical formulae for simferite:
These two recalculated analyses indicate that significant amounts of Mg2+ occur on the M2 site and that lithium is higher than 0.5 Li pfu on the M1 site. According to the dominant-valency rule (Hatert and Burke, 2008), the end-member formula of simferite should show Li dominant on M1 and divalent cations dominant on M2, among which Mg is the most abundant element.
We consequently revised the end-member formula of simferite (Table 2) to LiMg(PO4) in order to be consistent with the nomenclature of the triphylite group in which end-member formulae are expressed with one (PO4) group per formula unit.
It is important to note that the compound LiMg(PO4) exists as a synthetic product and has been extensively investigated, since it is used as an efficient phosphor (Gai et al., 2013; Marczewska et al., 2016; Kulig et al., 2017).
The new CNMNC-approved end-member formulae of minerals of the triphylite group are given in Table 2 (Miyawaki et al., 2022).
According to traditional views, a change in mineral species during oxidation (Quensel–Mason sequence) occurs in the triphylite → ferrisicklerite → heterosite and lithiophilite → sicklerite → purpurite series; this corresponds to a displacement along horizontal arrows in Fig. 4a during simultaneous oxidation of iron and manganese.
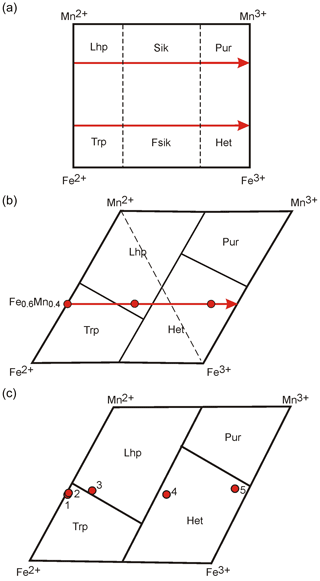
Figure 4Change in mineral species during oxidation of various original compositions. (a) Traditional view on the change in mineral species in the Quensel–Mason sequence. Elements located in vertices of the rectangle emphasize the view on simultaneous oxidation of iron and manganese. (b) Change in the original triphylite under partial oxidation to lithiophilite and complete oxidation to heterosite in the diagram Fe2+–Mn2+–Mn3+–Fe3+ where boundaries between minerals species are in accordance with the IMA rules. A shift in the line Mn2+–Mn3+ in relation to the line Fe2+-Fe3+ points out that iron oxidizes before manganese. (c) Triphylite series from Varuträsk (Quensel, 1937) at the diagram. Data are presented in the Supplement. Points: 1 – fresh blue triphylite; 2 – wine-yellow triphylite; 3 – somewhat oxidized triphylite; 4 – ferrisicklerite, with outer ring surrounding the fresh triphylite; and 5 – heterosite.
By considering the boundaries between mineral species, defined in accordance with the dominant-constituent and dominant-valency rules, we can observe that such a displacement of mineral compositions in certain areas of intermediate Fe–Mn compositions leads to a transition of triphylite under partial oxidation first into lithiophilite and then into heterosite (Fig. 4b). The accurate sequence is FeMn (triphylite) → MnFeFe (lithiophilite) → FeMn (heterosite). Triphylite may directly oxidize into heterosite when the manganese content in it is lower than 25 %. We can also show this feature by choosing as an example the chemical composition of triphylite analyzed by Quensel (1937) (see analysis of triphylite 3 in the Supplement and point 3 in Fig. 4c), which enters the lithiophilite field during oxidation.
The reason for this unexpected behavior is the different oxidation capacity (oxidation potential) of manganese and iron. During the oxidation of minerals of the triphylite–lithiophilite series with any ratio of components, iron oxidizes first. Consequently, in products of oxidation of minerals with different values of , i.e., with different contents of total iron (= initially different mineral species), the amount of Fe3+ can finally be similar. Then, newly formed phases will be related to one mineral species (Fig. 5). Thus, mineral phases with values > 0.5 cannot always be considered to represent iron end-members (triphylite–ferrisicklerite–heterosite), and those with values < 0.5 are not always manganese end-members (lithiophilite–sicklerite–purpurite).
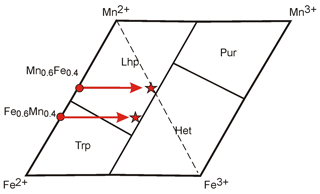
Figure 5Example of mineral species change under oxidation of minerals with a different ratio of iron and manganese in the diagram: Fe2+-Mn2+-Mn3+-Fe3+.
Consequently, the problem can only be solved by discarding the traditional views on succession of mineral species during oxidation. In other words, it is necessary to separate the concepts of the origin of the mineral and the boundaries of the species, and it should be recognized that the paragenetic relations between minerals cannot be connected in any way with an unambiguously defined formula for the species. Resolution of this rigid contradiction is rather distressing but essential.
The contradictory history goes back to the past when authors devoted little attention to the fact that despite being crystal chemically similar, manganese and iron have significantly different oxidation potentials. Indeed, if manganese and iron would oxidize more or less simultaneously, the problem with formulae would be non-existent; i.e., a cation prevailing in an original mineral in the divalent form would also prevail in its successor in the trivalent form. For that reason, the simple Quensel–Mason sequence was created and is still often used in publications. Starting from Fontan et al. (1976), differences in the behavior of iron and manganese were taken into account, and along with this comprehension, the confusion with formulae appeared. It is not obvious when a composition is mainly ferric or mainly manganoan; however, it is intractable for intermediate compositions.
As shown in Sect. 2, it is difficult to identify the triphylite-group minerals based on common available methods for chemical composition. It is clear that wet chemical methods using substance quantities are not suitable, taking into account the material heterogeneity due to, among other things, the well-known process of triphylite and lithiophilite oxidation. It is also evident that the most commonly used local microprobe analysis (EPMA) does not provide necessary data for the calculation in the case of Li-containing minerals. However, it is sufficient to additionally define lithium using one of the methods and make assumptions that iron oxidizes first; i.e., Mn3+ is introduced in the formula only when Fe2+ is completely absent.
Microprobe analyses provide data on the contents of Fetotal and Mntotal without dividing them into +2 and +3 valency states and provide no data on the lithium content. As a result, if we do not consider Mg, Na, and other minor elements, we obtain five unknown variables, i.e., Li, Fe2+, Mn2+, Fe3+, and Mn3+, two pairs of which are connected by equations
We obtain one of the unknown variables – Li content – by measuring it directly or, if such opportunity is absent, from single-crystal X-ray diffraction (SCXRD) data:
The total content of trivalent cations R3+ = (Fe3+ + Mn3+) in the M2 site is taken to be equal to
Based on these relations and assuming condition (5) of gradual rather than simultaneous oxidation of the elements, we get an amount of Fe2+, Mn2+, Fe3+, and Mn3+. The gradual oxidations mean that iron oxidizes first and completely. Manganese starts oxidizing only when all iron have transitioned into the oxidizing (Fe3+) state (see Sect. 4).
For convenience the calculation procedure for Li-containing minerals is given in Algorithm 1.
The examples of the calculation according to proposed procedures are presented in Table 3.
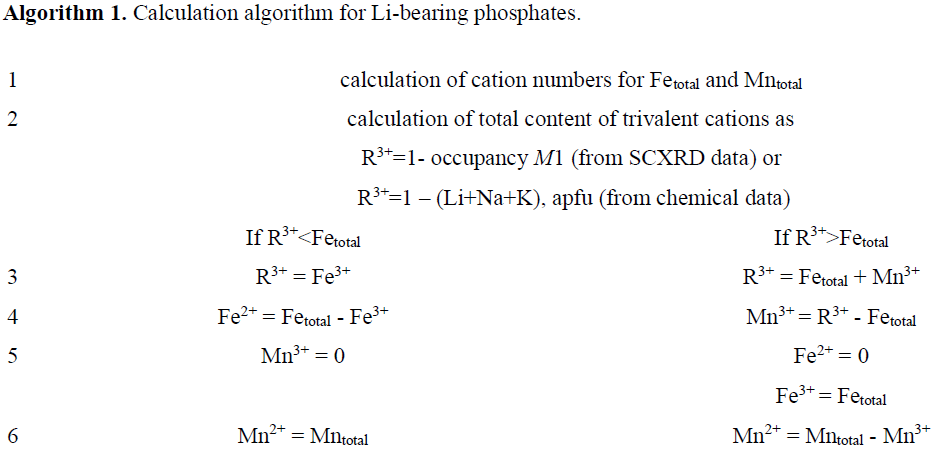
Table 3Calculation of trivalent cations and lithium contents according to proposed algorithm.
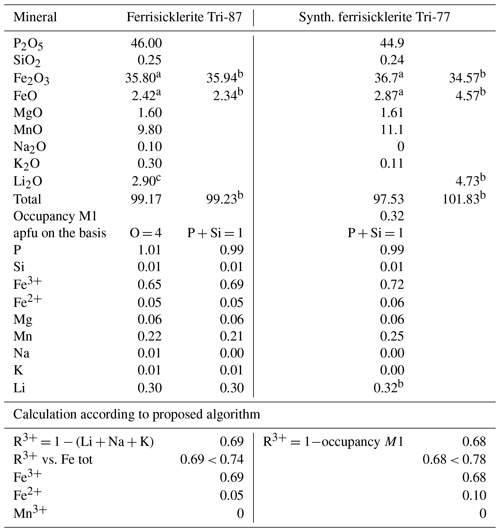
Ferrisicklerite Tri-87 and synth. ferrisicklerite Tri-77 from Schmid-Beurmann et al. (2013). These compositions correspond to heterosite according to proposed classification. a Determined by Mössbauer spectroscopy. b Calculated according to proposed algorithm. c Determined by SIMS.
All data derived from this research are available upon request from the corresponding author.
The supplement related to this article is available online at: https://doi.org/10.5194/ejm-35-427-2023-supplement.
LML initiated the project. All authors participated in the development of the concepts and the writing of the manuscript.
The contact author has declared that none of the authors has any competing interests.
Publisher's note: Copernicus Publications remains neutral with regard to jurisdictional claims in published maps and institutional affiliations.
This article is part of the special issue “New minerals: EJM support”. It is not associated with a conference.
We are grateful to Fernando Cámara and the anonymous reviewer for their careful reviews of this paper.
This work was funded by the Ministry of Science and Higher Education of the Russian Federation, project no. AAAA-A19-119100290149-1. Publisher's note: the article processing charges for this publication were not paid by a Russian or Belarusian institution.
This paper was edited by Sergey Krivovichev and Cristiano Ferraris, and reviewed by Fernando Cámara and one anonymous referee.
Alberti, A.: Crystal structure of ferrisicklerite, Li<1(Fe3+,Mn2+)PO4, Acta Crystallogr. B, 32, 2761–2764, 1976.
Alluaud, F.: Notices sur l'hétérosite, l'hureaulite (fer et manganèse phosphatés), et sur quelques autres minéraux du département de la Haute-Vienne, Anneles des Sciences Naturelles, 8, 334–354, 1826.
Baijot, M., Hatert, F., and Philippo, S.: Mineralogy and geochemistry of phosphates and silicates in the Sapucaia pegmatite, Minas Gerais, Brazil: genetic implications, Can. Mineral., 50, 1531–1554, https://doi.org/10.3749/canmin.50.6.1531, 2012.
Bayrakov, V. V., Yakubovich, O. V., Simonov, M. A., Borisovskiy, S. E., and Ziborova, T. A.: Simferite, Li(Mg,Fe3+, Mn3+)2[PO4]2, a new mineral, Mineral Zh., 27, 112–120, 2005.
Björling, C. O. and Westgren, A.: Minerals of the Varuträsk Pegmatite, Geol. Fören. Stock. För., 60, 67–72, 1938.
Brush, G. J. and Dana, E. S.: On a new and remarkable mineral locality in Fairfield County, Connecticut; with a description of several new species occurring there. First Paper, American Journal of Science and Arts, 116, 114–123, 1878.
Brush, G. J., Dana, E. S., and Wells, H. L.: On the mineral locality at Branchville, Connecticut. Fifth paper, Am. J. Sci., 39, 201–216, 1890.
Chapman, C. A.: Large magnesia-rich triphylite crystals in pegmatite, Am. Mineral., 28, 90–98, 1943.
Ercit, T. S., Piilonen, P. C., Locock, A. J., Kolitsch, U., and Rowe, R.: New mineral names, Am. Mineral, 91, 1201–1209, 2006.
Eventoff, W., Martin, R., and Peacor D. R.: The crystal structure of heterosite, Am. Mineral, 57, 45–51, 1972.
Fehr, K. Y., Hochleitner, R., Schmidbauer, E., and Schneider, J.: Mineralogy, Mössbauer spectra and electrical conductivity of triphylite Li(Fe2+,Mn2+)PO4, Phys. Chem. Miner., 34, 485–494, https://doi.org/10.1007/s00269-007-0164-8, 2007.
Finger, L. W. and Rapp, G. R.: Refinement of the crystal structure of triphylite, Carnegie I. Wash., 68, 290–292, 1969.
Fleischer's Glossary of Mineral Species 2008: 10th Edn., edited by: Back, M. E. and Mandarino, J. A., Mineralogical Record Inc., Tucson, Arizona 85740, USA, 346 pp., 2008.
Fontan, P. F., Huvelin, P., Orliac, M., and Permingeat, F.: La ferrisicklérite des pegmatites de Sidi Bou Othmane (Jebilet, Maroc) et le groupe des minéraux à structure de triphylite, B. Soc. Fr. Minéral. Cr., 99, 274–286, 1976 (in French with English abstract).
Fransolet, A. M., Antenucci, D., Speetjens, J. M., and Tarte, P.: An X-ray determinative method for the divalent cation ratio in the triphylite-lithiophilite series, Mineral. Mag., 48, 373–381, 1984.
Fransolet, A. M., Keller, P., and Fontan, F.: The phosphate mineral associations of the Tsaobismund pegmatite, Namibia, Contrib. Mineral. Petrol., 92, 502–517, 1986.
Fuchs, J. N.: Ueber ein neues Mineral (Triphylin), J. Prakt. Chem., 3, 98–104, 1834.
Gai, M., Chen, Z., Fan, Z., and Wang, J.: Synthesis and luminescence in LiMgPO4 : Tb,Sm,B phosphors with possible applications in real-time dosimetry, J. Rare Earths, 31, 551–554, 2013.
Graton, L. C. and Schaller, W. T.: Purpurite, a new mineral, Am. J. Sci., 20, 146–151, 1905.
Hatert, F.: Iron-Manganese Phosphates with the Olivine – and Alluaudite-Type Structures: Crystal Chemistry and Applications, in: Minerals as Advanced Materials II, edited by: Krivovichev, S., Springer Heidelberg, New York, Dordrecht, London, 279–291, https://doi.org/10.1007/978-3-642-20018-2, 2012.
Hatert, F. and Burke, E.: THE IMA–CNMNC dominant-constituent rule revisited and extended, Can. Mineral., 46, 717–728, https://doi.org/10.3749/canmin.46.3.717, 2008.
Hatert, F., Fransolet, A. M., and Maresch, W. V.: The stability of primary alluaudites in granitic pegmatites: an experimental investigation of the Na2(Mn2−2xFe)(PO4)3 system, Contrib. Mineral. Petr., 152, 399–419, https://doi.org/10.1007/s00410-006-0115-2, 2006.
Hatert, F., Ottolini, L., and Schmid-Beurmann, P.: Experimental investigation of the alluaudite + triphylite assemblage, and development of the Na-in-triphylite geothermometer: applications to natural pegmatite phosphates, Contrib. Mineral. Petr., 161, 531–546, https://doi.org/10.1007/s00410-010-0547-6, 2011a.
Hatert, F., Ottolini, L., Fontan, F., Keller, P., Roda-Robles, E., and Fransolet, A. M.: The crystal chemistry of olivine-type phosphates, the Contributions to the 5th International Symposium on Granitic pegmatites, 20–27 April 2011, Mendoza, Argentina, Associacin Geolgica Argentina, serie D, Publicacin Especial, 14, 103–105, 2011b.
Hatert, F., Ottolini, L., Wouters, J., and Fontan, F.: A structural study of the lithiophilite-sicklerite series, Can. Mineral., 50, 843–854, https://doi.org/10.3749/canmin.50.4.843, 2012.
Jana, S., Lingannan, G., Ishtiyak, M., Panigrahi, G., Sonachalam, A., and Prakash, J.: Syntheses, crystal structures, optical, Raman spectroscopy, and magnetic properties of two polymorphs of NaMnPO4, Mater. Res. Bull., 126, 110835, https://doi.org/10.1016/j.materresbull.2020.110835, 2020.
Kulig, D., Gieszczyk, W., Marczewska, B., Bilski, P., Kłosowski, M., and Malthez, A. L. M. C.: Comparative studies on OSL properties of LiMgPO4 : Tb,B powders and crystals, Radiat. Meas., 106, 94–99, 2017.
Langer, K., Taran, M. N., and Fransolet, A. M.: Electronic absorption spectra of phosphate minerals with olivine-type Structures: II. The oxidized minerals ferrisicklerite, Lix)M2[6](FeMn)[PO4], and heterosite, M1[6](□1.00)M2[6](FeMn)[PO4], with x≤0.5, Eur. J. Mineral., 19, 589–592, https://doi.org/10.1127/0935-1221/2007/0019-1742, 2007.
Le Page, Y. and Donnay, G.: The crystal structure of the new mineral marićite, NaFePO4, Can. Mineral., 15, 518–521, 1977.
Losey, A., Rakovan, J., Hughes, J. M., Francis, C. A., and Dyar, M. D.: Structural variation in the lithiophilite-triphylite series and other olivine-group structures, Can. Mineral., 42, 1105–1115, 2004.
Marczewska, B., Bilski, P., Wróbel, D., and Kłosowski, M.: Investigations of OSL properties of LiMgPO4 : Tb,B based dosimeters, Radiat. Meas., 90, 265–268, 2016.
Mills, S. J., Hatert, F., Nickel, E. H., and Ferraris, G.: The standardisation of mineral group hierarchies: application to recent nomenclature proposals, Eur. J. Mineral., 21, 1073–1080, https://doi.org/10.1127/0935-1221/2009/0021-1994, 2009.
Miyawaki, R., Hatert, F., Pasero, M., and Mills, S. J.: IMA Commission on New Minerals, Nomenclature and Classification (CNMNC) – Newsletter 70, Eur. J. Mineral., 34, 591–601, https://doi.org/10.5194/ejm-34-591-2022, 2022.
Moore, P. B.: Natrophilite, NaMn(PO4), has ordered cations, Am. Mineral., 57, 1333–1344, 1972.
Padhi, A. K., Nanjundaswamy, K. S., and Goodenough J. B.: Phospho-olivines as positive materials for rechargeable lithium batteries, J. Electrochem. Soc., 144, 1188–1194, 1997.
Quensel, P.: Minerals of the Varuträsk Pegmatite. I. The lithium – manganese phosphates, Geol. Fören. Stock. För., 59, 77–96, 1937.
Rakovan, J.: Li-Phosphate Minerals and Storage Batteries, Mineral Maters Column in Elements, 1, 125, 2005.
Roda-Robles, E., Pesquera, A., García de Madinabeitia, S., Gil Ibarguchi, J., Nizamoff, J., Simmons, W., Falster, A., and Galliski, M. A.: On the geochemical character of primary Fe-Mn phosphates belonging to the triphylite-lithiophilite, graftonite-beusite, and triplite-zwieselite series: first results and implications for pegmatite petrogenesis, Can. Mineral., 52, 321–335, https://doi.org/10.3749/canmin.52.2.321, 2014.
Schaller, W. T.: New manganese phosphates from the gem tourmaline field of Southern California, Journal of the Washington Academy of Sciences, 2, 143–145, 1912.
Schmid-Beurmann, P., Ottolini, L., Hatert, F., Geisler, T., Huyskens, M., and Kahlenberg, V.: Topotactic formation of ferrisicklerite from natural triphylite under hydrothermal conditions, Miner. Petrol., 107, 501–515, https://doi.org/10.1007/s00710-012-0250-6, 2013.
Shannon, R. D., Shannon, R. C., Medenbach, O., and Fischer, R. X.: Refractive Index and Dispersion of Fluorides and Oxides, J. Phys. Chem. Ref. Data, 31, 931–970, 2002.
Shigley, J. E. and Brown, G. E.: Occurence and alteration of phosphate minerals at the Stewart Pegmatite, Pala District, San Diego County, California, Am. Mineral., 70, 395–408, 1985.
Struman, B. D., Mandarino, J. A., and Corlett, M. I.: Marićite, a sodium iron phosphate, from the Big Fish River area, Yukon Territory, Canada, Can. Mineral., 15, 396–398, 1977.
Svetov, S. A., Stepanova, A. V., Chazhengina, S. Y., Svetova, E. N., Rybnikova, Z. P., Mikhailova, A. I., Paramonov, A. S., Utitsyna, V. L., Ekhova, M. V., and Kolodey, B. S.: Precision geochemical (ICP-MS, LA-ICP-MS) analysis of rock and mineral composition: the method and accuracy estimation in the case study of Early Precambrian mafic complexes, Proceedings of the Karelian Research Centre RAS, 7, 54–73, https://doi.org/10.17076/geo140, 2015.
Vignola, P., Hatert, F., Fransolet, A.-M., Medenbach, O., Diella, V., and Andò, S.: Karenwebberite, Na(Fe2+,Mn2+)PO4, a new member of the triphylite group from the Malpensata pegmatite, Lecco Province, Italy, Am. Mineral., 98, 767–772, 2013.
Yakubovich, O., Khasanova, N., and Antipov, E.: Mineral-Inspired Materials: Synthetic Phosphate Analogues for Battery Applications, Minerals, 10, 524, https://doi.org/10.3390/min10060524, 2020.
Yakubovich, O. V., Bairakov, V. V., and Simonov, M. A.: Crystal structure of simferite, Li(Mg,Fe3+,Mn3+)2[PO4]2, Proceedings of the Academy of Sciences USSR, 307, 1119–1122, 1989 (in Russian).
Yamada, A., Takei, Y., Koizumi, H., Sonoyama, N., Kanno, R., Itoh, K., Yonemura, M., and Kamiyama, T.: Electrochemical, magnetic, and structural investigation of the Lix(MnyFe1−y)PO4 olivine phases, Chem. Mater., 18, 804–813, https://doi.org/10.1021/cm051861f, 2006.
- Abstract
- Introduction
- Previous data on the triphylite group of phosphates
- Nomenclature problem
- The new classification scheme
- Mineral sequence during oxidation
- Calculation of empirical formulae
- Data availability
- Author contributions
- Competing interests
- Disclaimer
- Special issue statement
- Acknowledgements
- Financial support
- Review statement
- References
- Supplement
- Abstract
- Introduction
- Previous data on the triphylite group of phosphates
- Nomenclature problem
- The new classification scheme
- Mineral sequence during oxidation
- Calculation of empirical formulae
- Data availability
- Author contributions
- Competing interests
- Disclaimer
- Special issue statement
- Acknowledgements
- Financial support
- Review statement
- References
- Supplement