the Creative Commons Attribution 4.0 License.
the Creative Commons Attribution 4.0 License.
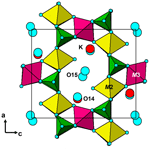
Pleysteinite, [(H2O)0.5K0.5]2Mn2Al3(PO4)4F2(H2O)10 ⋅ 4H2O, the Al analogue of benyacarite, from the Hagendorf-Süd pegmatite, Oberpfalz, Bavaria, Germany
Ian E. Grey
Rupert Hochleitner
Christian Rewitzer
Anthony R. Kampf
Colin M. MacRae
Robert W. Gable
William G. Mumme
Erich Keck
Cameron Davidson
Pleysteinite, [(H2O)0.5K0.5]2Mn2Al3(PO4)4F2(H2O)10 • 4H2O, is the aluminium analogue of benyacarite, from the Hagendorf-Süd pegmatite, Oberpfalz, Bavaria, Germany. It was found in specimens of altered zwieselite, in association with nordgauite, fluellite, rockbridgeite, pyrite and columbite. Pleysteinite occurs as isolated and small aggregates of colourless, stubby prisms that are typically 10 to 30 µm wide and up to 100 µm long. The crystals are flattened on {010} and bounded by {111}, {100} and {001} planes. The calculated density is 2.34 g cm−3. Optically, pleysteinite crystals are biaxial (+), with α=1.566(2), β=1.580(2), γ=1.600(2) (measured in white light) and 2V(meas.) = 80(1)∘. The empirical formula from electron microprobe analyses and structure refinement is [(H2O)0.50K0.50]2(Mn1.20Mg0.49FeZn0.05)∑2.01(Al1.63FeTi)∑2.02(Al0.56Ti) (PO4)4.02[F0.58O0.31(OH)0.11]2(H2O)10 • 3.92H2O. Pleysteinite has orthorhombic symmetry, with space group Pbca and unit-cell parameters a = 10.4133(8) Å, b=20.5242(17) Å, c=12.2651(13) Å, V=2621.4(4) Å3 and Z=4. The crystal structure was refined using single-crystal data to wRobs=0.054 for 1692 reflections with I>3σ(I). The crystal structure contains corner-connected linear trimers of Al-centred octahedra that share corners with PO4 tetrahedra to form 10-member rings parallel to (010). K+ cations and water molecules are located in the rings. Additional corner-sharing of the PO4 tetrahedra with Mn(H2O)4O2 octahedra occurs along [010] to complete the 3D framework structure.
- Article
(6614 KB) - Full-text XML
-
Supplement
(252 KB) - BibTeX
- EndNote
Pleysteinite was found in specimens of altered zwieselite from the Hagendorf-Süd pegmatite mine, Bavaria. The specimens were collected in the 1970s by one of the authors (Erich Keck) from the 64–67 m level of the mine. Energy-dispersive spectroscopic (EDS) analysis of the small prismatic crystals of the mineral indicated that it was most likely the aluminium analogue of the titanium-bearing phosphate mineral benyacarite, (H2O,K)2(Mn2+,Fe2+)2(Fe3+,Ti)2Ti(PO4)4(O,F)2• 14H2O (Demartin et al., 1993, 1997), and this was confirmed from a single-crystal structure determination.
Benyacarite is closely related to two other titanium-bearing phosphate minerals, paulkerrite, KTi(Mg,Mn)2(Fe3+,Mg,Al,Ti)2(PO4)4(OH)3 • 15H2O (Peacor et al., 1984) and mantienneite, (K0.5□0.5)Ti(Mg1.5Fe)∑2Al2(PO4)4(OH)3 • 15H2O, □ = vacancy (Fransolet et al., 1984). Demartin et al. (1993) proposed the general formula (H2O, K)2TiM12M22(PO4)4(O,F)2 • 14H2O for these minerals, which are distinguished by the dominant cations at the M1 and M2 sites: Mn2+ and Fe3+ in benyacarite, Mg and Fe3+ in paulkerrite and Mg and Al in mantienneite. A proposal for a paulkerrite group, based on the first-described member, has been submitted to the International Mineralogical Association (IMA) Commission on New Minerals, Nomenclature and Classification (CNMNC). Pleysteinite is the fourth member of the group, with dominant Mn2+ at the M1 site and dominant Al at the M2 and Ti sites. Full characterisation of the mineral led to its approval as a new species by the IMA CNMNC, proposal IMA2022-077. The name is for Pleystein, a municipality in Bavaria located 18 km east of Weiden in the Oberpfalz. The Hagendorf-Süd pegmatite that provided the specimens of pleysteinite is situated near the municipal area of Pleystein. The holotype specimen is housed in the collections of the Mineralogical State Collection Munich (SNSB), with registration number MSM 38032. A cotype used for optical characterisation and the powder diffraction pattern is housed in the mineralogical collections of the Natural History Museum of Los Angeles County, catalogue number 76271.
The holotype specimen, Hag500, containing pleysteinite crystals on altered zwieselite (Fig. 1) comes from the Hagendorf-Süd pegmatite, Oberpfalz, Bavaria, Germany (49∘39′1′′ N, 12∘27′35′′ E). The zwieselite specimens are bleached to a very light maroon colour and contain many vugs up to some millimetres in diameter. The fine-grained zwieselite is very soft and sometimes shows a silky lustre. The larger holes contain rockbridgeite, Fe2+Fe(PO4)3(OH)5, and crystals of fluellite, Al2(PO4)F2OH • 7H2O, up to 1 mm. The smaller vugs contain silky needles of white nordgauite, MnAl2(PO4)2(F,OH)2 • 5H2O, and are lined with colourless equant crystals of pleysteinite up to 0.1 mm. Nordgauite is younger than pleysteinite. Embedded and sometimes protruding into vugs are cubes of pyrite and tiny tabular crystals of columbite which are older than the zwieselite.
Pleysteinite forms isolated and small aggregates of colourless, stubby prisms on fine-grained zwieselite (Fig. 1). The crystals are typically 10 to 30 µm wide and up to 100 µm long and often display pyramidal terminations (Fig. 2). A feature of the crystals is that they readily cleave into thin tablets parallel to {010} in a vacuum due to dehydration (Fig. 3). The crystals are flattened on {010} and bounded by {111}, {100} and {001} planes (Fig. 2). The calculated density, for the empirical formula and single-crystal unit-cell volume, is 2.34 g cm−3.
Optically, pleysteinite crystals are biaxial (+), with α=1.566(2), β=1.580(2), γ=1.600(2) (measured in white light). The measured 2V from extinction data analysed with EXCALIBR (Gunter et al., 2004) is 80(1)∘, and the calculated 2V is 80.7∘. Dispersion and pleochroism were not observed. The optical orientation is X=c, Y=b, Z=a. The Gladstone–Dale compatibility index (Mandarino, 1981) is −0.019 (superior) based on the empirical formula and the calculated density.
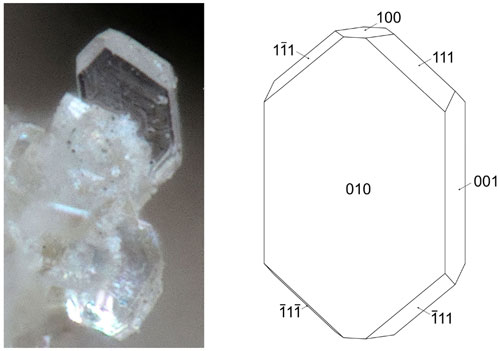
Figure 2Pleysteinite crystal (photo Christian Rewitzer) with crystal drawing (clinographic projection in nonstandard orientation, a vertical) showing face indexing.
Crystals of pleysteinite were analysed using wavelength-dispersive spectrometry on a JEOL JXA-8500F Hyperprobe operated at an accelerating voltage of 15 kV and a beam current of 2.2 nA. The beam was defocused to 10 µm. Analytical results (average of analyses on seven crystals) are given in Table 1. There was insufficient material for direct determination of H2O, so it was based upon the crystal structure analysis. The atomic proportions, normalised to 33 anions and with based on the crystal structure ((Fe2+ with other divalent cations at the M1 site and remaining iron as Fe3+ at the M2 site), are K1.00Mn1.20Mg0.49FeZn0.05Al2.19FeTiP4.02F1.16O31.84H30.06.
The empirical formula is calculated based on the crystal structure, with divalent cations located at M1 and with the site assignment at M3 from the site occupancy refinement, and the remaining small cations from the EMP analyses located at M2: [(H2O)0.5K0.5]2M1(Mn1.20Mg0.49FeZn0.05)∑2.01M2(Al1.63FeTi)∑2.02M3(Al0.56Ti)(PO4)4.02[F0.58O0.31(OH)0.11]2(H2O)10 • 3.92H2O.
The simplified formula is [(H2O)0.5K0.5]2(Mn,Mg,Fe2+,Zn)2(Al,Fe3+,Ti4+)2(Al,Ti4+)(PO4)4(F,O,OH)2(H2O)10• 4H2O.
The ideal formula is [(H2O)0.5K0.5]2Mn2Al3(PO4)4F2(H2O)10 • 4H2O, which requires K2O 5.13, MnO 15.46, Al2O3 16.66, P2O5 30.93, F 4.14, H2O 29.42, -O≡F −1.74, total 100 wt %. Note that the •14H2O in the Demartin et al. (1993) general formula has been separated into (H2O)10 coordinated at the M1 and M2 sites and •4H2O molecules occupying cavities, to better reflect the crystal structure.
Raman spectroscopy was conducted on a Horiba XploRA PLUS spectrometer using a 532 nm diode laser, 100 µm slit and 1800 g mm−1 diffraction grating and a 100× (0.9 NA) objective. The spectrum is shown in Fig. 4. The O–H stretch region has a broad peak that can be assigned to H-bonded water, with a maximum at 3335 cm−1 and a shoulder at 3115 cm−1. A sharp shoulder at 3560 cm−1 is most likely due to hydroxyls. The H–O–H bending mode region for water has a peak at 1665 cm−1. The P–O stretching region has strong sharp peaks at 960 and 1015 cm−1 and a weaker peak at 1130 cm−1 with a shoulder at 1095 cm−1. The two strong peaks correspond to antisymmetric stretching modes, and the weaker peaks correspond to symmetric P–O stretching modes. Bending modes of the (PO4)3− groups are manifested by two bands centred at 610 and 460 cm−1. Peaks at lower wavenumbers are related to lattice vibrations. A strong peak at 850 cm−1 with a shoulder at 780 cm−1 is an interesting feature of the spectrum. A tentative assignment is to stretching vibrations for short M–(O,F) bonds that occur in linear trimers of corner-connected octahedra M2–M3–M2 in the structure (Fig. 5). The potassium titanyl phosphate, KTiOPO4, has chains of corner-connected octahedra with short (1.81–1.83 Å)Ti–O bonds, and the Raman spectrum has an intense band at 770 cm−1 that has been assigned to the symmetric Ti–O stretching vibration (Tu et al., 1996). Strong Raman bands in the range 800 to 900 cm−1 have been reported and assigned to Ti–O stretch vibrations for several potassium titanates, which have corner-connected TiO6 octahedra involving short (∼ 1.8 A) bridging Ti–O bonds (Bamberger et al., 1990). The M2 site in pleysteinite similarly has the M2 atom displaced from the centre of the octahedron towards the corner-sharing anion with the M3 octahedron giving a short (1.825 A) M2–(O,F) bond.
X-ray powder diffraction data were recorded using a Rigaku R-Axis Rapid II curved imaging plate microdiffractometer with monochromatised Mo Kα radiation. A Gandolfi-like motion on the ϕ and ω axes was used to randomise the sample. Observed d values and intensities were derived by profile fitting using JADE Pro software (Materials Data, Inc.). Data are given in Table 2. Refined orthorhombic unit-cell parameters (space group Pbca (#61)) are a=10.407(4) Å, b=20.557(8) Å, c=12.247(5) Å, V=2619.9(7) Å3 and Z=4.
Single-crystal diffraction data were collected at room temperature using a Rigaku Oxford Diffraction Supernova four-circle diffractometer equipped with an Atlas CCD detector and using MoKα radiation (λ=0.71073 Å). Refined unit-cell parameters and other data collection details are given in Table 3.
Structure refinement
A structural model was obtained in space group Pbca using SHELXT (Sheldrick, 2015). It was found to be the same as that reported for benyacarite (Demartin et al., 1993). The scattering curve for Mn was used to represent (Mn + Mg + Fe + Zn) at the M1 site, and Fe and Al were located at the M2 site with their occupancies refined. The K + O (for H2O) occupancies at the K site were also refined. The mean bond distance for the Ti site (≡M3) was found to be only 1.91 Å, which is too short for Ti4+–O (expected 1.96 Å from bond valence sum (BVS) for an ideal octahedron), and the isotropic displacement parameter was elevated (U=0.03), suggesting replacement by a lighter cation. Al was added to the M3 site and the occupancies of Al/Ti refined, giving dominant Al in the site [Al0.56(1)Ti0.44(1)].
Table 4Atom coordinates, equivalent isotropic displacement parameters (Å2) and bond-valence sums (BVSs) for pleysteinite.
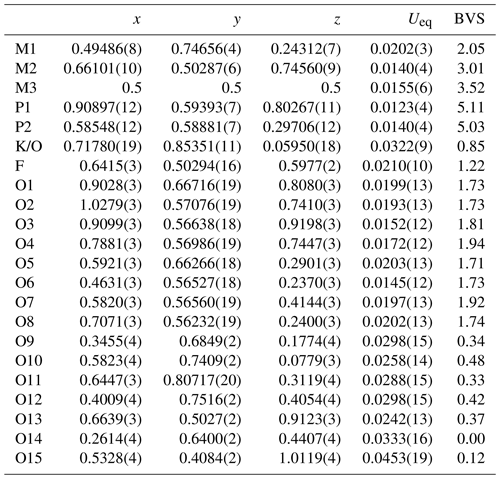
Site scattering: M1 = 0.950(4)Mn; M2 = 0.685(6)Al+0.315Fe; M3 = 0.56(1)Al + 0.44Ti; K/O = 0.473(9)K + 0.527O.
Refinement with anisotropic displacement parameters for all atoms in JANA2006 (Petříček et al., 2014) converged at wRobs=0.054 for 1692 reflections with I>3σ(I). Unambiguous location of H atoms in correct configurations for H2O molecules in difference Fourier maps was not achieved. Partial H positions have been previously reported for benyacarite (Demartin et al., 1993). Details of the data collection and refinement are given in Table 3. The refined coordinates, equivalent isotropic displacement parameters and bond valence sum (BVS) values (Gagné and Hawthorne, 2015) from the single-crystal refinement are reported in Table 4. Selected interatomic distances are reported in Table 5. Although the H atoms were not located, the BVS values in Table 4 show clearly the presence of seven independent H2O molecules, O9 to O15. An indication of H2O at the K/O site was obtained by pairs of weak peaks in a difference Fourier map at 0.8 Å from the central atom and making a H–O–H angle of 100∘.
The structure comprises [100] chains of alternating corner-connected M2O4F(H2O) octahedra and PO4 tetrahedra that are connected along [001] by corner-sharing with M3O4F2 octahedra into (010) layers shown in Fig. 5. The corner-connected polyhedra form 10-member rings that are occupied by K+ ions and water molecules, O14 and O15. These layers are interconnected into a 3D framework structure via corner sharing of the PO4 tetrahedra with M1O2(H2O)4 octahedra, shown in Fig. 6. The K site contains approximately equal amounts of K+ ions and H2O molecules, as found also for benyacarite (Demartin et al., 1993). The coordination is irregular, with six distances in the range 2.72 to 3.09 Å (Table 5) and two longer distances at ∼ 3.3 Å.
Pleysteinite is isostructural with benyacarite (Demartin et al., 1993). The published orthorhombic unit-cell parameters for paulkerrite and mantienneite suggest that these two minerals also have the same crystal structure as benyacarite. Demartin et al. (1997) proposed a general formula for the three minerals, (H2O,K)2TiM12M22(PO4)4(O,F)2 • 14H2O, with divalent cations, Mn2+ and Mg2+ at the M1 site and trivalent cations, Fe3+ and Al3+ at the M2 site. All three minerals have approximately one Ti4+ cation per formula unit, and this was allocated to the special site (1/2,1/2,0) in Pbca in the refinement of benyacarite. Our refinement of the pleysteinite crystal structure, however, shows that Al, rather than Ti, is dominant at the Ti site, and the Ti is partitioned between the Ti and M2 sites. On this basis the formula proposed by Demartin et al. (1997) needs modifying to replace the Ti site with a more general M3 site. We have also separated the •14H2O in the Demartin formula into (H2O)10 coordinated at the M1 and M2 sites and •4H2O molecules occupying cavities, to better reflect the crystal structure. The resulting formula is A2M12M22M3(PO4)4X2(H2O)10 • 4H2O, where A = K, H2O and X = O, F, OH.
Crystallographic data for pleysteinite are available in the Supplement.
The supplement related to this article is available online at: https://doi.org/10.5194/ejm-35-189-2023-supplement.
IEG oversaw the research and wrote the paper. EK was responsible for the field collection of the specimen. RH and CR obtained preliminary EDS analyses, and CR obtained optical images of the specimen. WGM assisted in the diffraction data analysis. CMM conducted the EMP analyses. ARK measured the optical properties, Raman spectrum, PXRD and crystal morphology. RWG collected the single-crystal diffraction data, and CD prepared polished mounts of the specimen for EMP analyses.
The contact author has declared that none of the authors has any competing interests.
Publisher’s note: Copernicus Publications remains neutral with regard to jurisdictional claims in published maps and institutional affiliations.
We thank Matt Glenn for obtaining scanning electron microscopy data, including Fig. 3.
This paper was edited by Sergey Krivovichev and reviewed by two anonymous referees.
Bamberger, C. E., Begun, G. M., and MacDougall, C. S.: Raman spectroscopy of potassium titanates: Their synthesis, hydrolytic reactions and thermal stability, Appl. Spectrosc., 44, 31–37, 1990.
Demartin, F., Pilati, T., Gay, H. D., and Gramaccioli, C. M.: The crystal structure of a mineral related to paulkerrite, Z. Kristallogr., 208, 57–71, 1993.
Demartin, F., Gay, H. D. Gramaccioli, C. M., and Pilati, T.: Benyacarite, a new titanium-bearing phosphate mineral species from Cerro Blanco, Argentina, Can. Mineral., 35, 707–712, 1997.
Dowty, E.: ATOMS, Shape Software, Kingsport, Tennessee, USA, 2006.
Fransolet, A.-M., Oustriere, P., Fontan, F., and Pillard, F.: La mantiennéite, une novelle espèce minérale du gisement de vivianite d'Anloua, Cameroun. Bull. Mineral., 107, 737–744, 1984.
Gagné, O. C. and Hawthorne, F. C.: Comprehensive derivation of bond-valence parameters for ion pairs involving oxygen, Acta Crystallogr. B, 71, 562–578, 2015.
Gunter, M. E., Bandli, B. R., Bloss, F. D., Evans, S. H., Su, S. C., and Weaver, R.: Results from a McCrone spindle stage short course, a new version of EXCALIBR, and how to build a spindle stage, Microscope, 52, 23–39, 2004.
Mandarino, J. A.: The Gladstone-Dale relationship: Part IV. The compatibility concept and its application, Can. Mineral., 19, 441–450, 1981.
Peacor, D. R., Dunn, P. J., and Simmons, W. B.: Paulkerrite a new titanium phosphate from Arizona, Mineral. Rec., 15, 303–306, 1984.
Petříček, V., Dušek, M., and Palatinus, L.: Crystallographic Computing System JANA2006: General features, Z. Kristallogr., 229, 345–352, 2014.
Sheldrick, G. M.: Crystal-structure refinement with SHELX, Acta Crystallogr. C, 71, 3–8, 2015.
Tu, C.-S., Guo, A. R., Tao, R., Katiyar, R. S., Guo, R., and Bhalla, A. S.: Temperature dependent Raman scattering in KTiOPO4 and KTiOAsO4 single crystals, J. Appl. Phys., 79, 3235–3240, 1996.