the Creative Commons Attribution 4.0 License.
the Creative Commons Attribution 4.0 License.
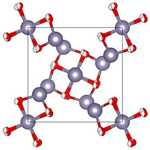
Tin weathering experiment set by nature for 300 years: natural crystals of the anthropogenic mineral hydroromarchite from Creussen, Bavaria, Germany
Natalia Dubrovinskaia
Maria Messingschlager
Leonid Dubrovinsky
Hydroromarchite is a mineral that so far has been found only in a few locations in the world and recognized as a common product of submarine corrosion of pewter artefacts. Here we report a new locality for this rare mineral found at the Saint James Church archaeological site in Creussen, Germany. There it appeared to be a product of weathering of a tin artefact (a tin button) buried in soil of the churchyard for about 300 years. The mineral, found in paragenesis with romarchite and cassiterite, was identified using single-crystal X-ray diffraction.
- Article
(14295 KB) - Full-text XML
- BibTeX
- EndNote
The rare mineral hydroromarchite is the natural occurrence of hydrous tin (II) oxide. It is approved by the International Mineralogical Association (IMA) and remains in the IMA List of Minerals providing the following information:
-
approved formula: SnO2(OH)2
-
IMA status: A (approved)
-
IMA no./year: 1969-007
-
country: Canada
-
first reference: Canadian Mineralogist 10 (1971), 916 (Organ and Mandarino, 1971)
-
second reference: Canadian Mineralogist 41 (2003), 649 (Ramik et al., 2003).
Organ and Mandarino (1971) claimed to find natural hydrous tin (II) oxide on tin artefacts in a freshwater environment, which they named hydroromarchite, on the basis of its powder X-ray diffraction (XRD) pattern matching that reported by Donaldson (1961) for synthetic crystalline hydrous tin (II) oxide. As the powder XRD pattern was not indexed (Donaldson, 1961), the first available crystallographic information was limited by the triclinic lattice parameters. The crystal data of Donaldson (1961) were superseded by the data from single-crystal X-ray diffraction (SCXRD) measurements, also on synthetic crystals (Howie and Moser, 1968), which revealed their tetragonal symmetry. Howie and Moser (1968) were the first who suggested a structure model for the tin (II) oxyhydroxide based on the Sn6O8 structural unit, consisting of an approximately regular cube of oxygen atoms (side 2.82 Å) superimposed on an octahedral cluster of tin atoms (side 3.59 Å). The following crystallographic data were reported: the unit cell parameters a=7.93(1) and c=9.13(1) Å; the space group (from systematic absences) P4/mnc or P4nc (Howie and Moser, 1968, 1973); the number of formula units (3SnO ⋅ H2O) per unit cell Z=4. The atomic coordinates were not provided in the papers, and the quality of the structural refinement was very moderate (R-factor equal to 16 %). A group of co-authors (Ramik et al., 2003), including the authors of the first report of hydroromarchite in 1971 (Organ and Mandarino, 1971), re-examined their powder XRD data and accepted tetragonal symmetry and the space group 21c (Ramik et al., 2003). This space group was suggested by Abrahams et al. (1996), who refined the structure of synthetic tin (II) oxyhydroxide (JCPDS file number 46-1486) by Rietveld analysis, using in the starting model the atomic parameters of the isostructural lead analogue (Hill, 1985). The unit cell parameters reported by Abrahams et al. (1996) are a=7.9268(4) and c=9.1025(5) Å.
The latest report on the low-temperature SCXRD of synthetic hydroromarchite appeared as a short abstract for a crystallographic meeting (Reuter and Schröder, 2010). High-quality and large enough single crystals were found by Reuter and Schröder (2010) by chance, in a more than 10-year-old sample of nBuSnH3 in toluene. The unit cell parameters and the space group (a=7.8809(2), c=9.0595(4) Å, and 21c) provided there (Reuter and Schröder, 2010) agree with those reported by Abrahams et al. (1996). Unfortunately, the abstract by Reuter and Schröder (2010) does not contain information about the atomic coordinates, atomic displacement parameters, and experimental temperature, and since then the complete data of the structure refinement have not been published or deposited to any crystallographic database. Thus, the reported structural data based on the SCXRD analysis are either incomplete and insufficiently accurate (Howie and Moser, 1968, 1973) or their details are unavailable (Reuter and Schröder, 2010). Moreover, they disagree with regard to the space group: Howie and Moser (1968) suggested either P4/mnc or P4nc, whereas Reuter and Schröder (2010) found it to be 21c, which matches the results of powder XRD analysis reported for both the synthetic samples (Abrahams et al., 1996) and mineral hydroromarchite (Organ and Mandarino, 1971; Ramik et al., 2003; Dunkle et al., 2003).
All researchers who have studied synthetic tin (II) oxyhydroxide have been concerned that the preparation of single crystals of this compound had proven to be particularly difficult. The controversy in the crystal symmetry could likely be resolved if single crystals of hydroromarchite could be found in nature and studied using modern methods of SCXRD analysis. That appeared to be the case in the present work. Here we report a new natural occurrence of the anthropogenic mineral hydroromarchite after only a few unambiguously confirmed instances so far (Organ and Mandarino, 1971; Ramik et al., 2003; Dunkle et al., 2003, 2004; Berger et al., 2019; Di Martino et al., 2019). It was found not in a water environment but in soil. The results of the hydroromarchite's structural investigation and its SCXRD data analysis are described below.
Hydroromarchite is a substance formed from man-made materials (tin or its alloys with a minor amount of alloying metals, Cu, Fe, As, and Pb, called pewter) and found mainly on archaeological artefacts. Since 1998 such substances have not been considered by the IMA Commission on New Minerals, Nomenclature and Classification (CNMNC) as minerals (Nickel and Grice, 1998; Hazen et al., 2017). The hydroromarchite, however, although not matching the current IMA CNMNC's definition of a mineral (Nickel, 1995), still preserves the “title”, as it was approved as a mineral a long time before. As suggested by Hazen and co-authors (Hazen et al., 2017), it should be considered among natural phases in the category “II. Minerals associated with archaeological artefacts” in the group “C. Alteration of tin artefacts” (Hazen et al., 2017). In this light hydroromarchite is certainly qualified to be called an anthropogenic mineral (also in light of its origin and locality identified in this work; see below) but qualifying it as a natural mineral (Hazen et al., 2017) based on its finding in Cantiere Speranza (Corchia mine), Italy (Garuti and Zaccarini, 2005), is likely not quite justified yet. The mineral from Corchia mine was identified only on the basis of its chemical composition deduced from results of several electron-microprobe analyses, and the authors Garuti and Zaccarini (2005) themselves wrote that “since no Sn-mineral precursor has ever been reported from the Northern Apennine copper deposits, a natural origin of the hydroromarchite from Corchia could be seriously challenged” and “…a complete characterization is needed to confirm its mineralogical nature” (Garuti and Zaccarini, 2005).
Hydroromarchite was found only in a few locations in the world and is recognized as a common product of submarine corrosion of pewter artefacts. Along with romarchite (SnO), it was first identified on tin pannikins, which were exposed to fresh water of Winnipeg River at Boundary Falls, Ontario, for about 150–170 years (Organ and Mandarino, 1971; Ramik et al., 2003). Then it was found on plates and other pewter artefacts (made of tin with a minor amount of alloying metals Cu, Fe, As, and Pb) recovered from the shipwreck Queen Anne's Revenge archaeological site (Beaufort, North Caroline) after being exposed to salty oceanic water for about 280 years (Dunkle et al., 2003). In both cases the identification was made on the basis of X-ray diffraction data. Dunkle et al. (2004) reported on a few other findings in submarine environments (see Dunkle et al., 2004, and references therein).
Finding hydroromarchite in a non-underwater environment is even rarer. During an excavation in the Mochlos settlement (Crete, Greece) in 2004, a tin ingot was unearthed, and some hydroromarchite could be identified among the products of the ingot's disintegration (Berger et al., 2019). Investigations of fragments of a tin-rich alloy organ pipe (mid-18th century), coming from an instrument placed in the church of San Giovanni Battista in Chieti (Abruzzo) in central Italy (Di Martino et al., 2019), revealed hydroromarchite among products of the organ pipe's corrosion due to moisture. Present work has added one more location to the currently quite short list due to finding hydroromarchite as a product of a tin artefact weathering in soil for about 300 years.
The tin artefact, whose alteration led to the formation of hydroromarchite, was found at an archaeological site in the old city of Creussen, located in the state of Bavaria, 12 km south of Bayreuth (Germany). The Bavarian cultural heritage preservation legislation (das Bayerische Denkmalschutzgesetz) has ensured that no valuable site will be destroyed by construction without study. The archaeological excavation in the area of the former cemetery both at the south and the north sides of Saint James Church (Fig. 1) (latitude: 49.843975/49∘50′38.31′′ N, longitude: 11.623794/11∘37′25.657′′ E) started in relation to the church's general renovation that began in 2017.
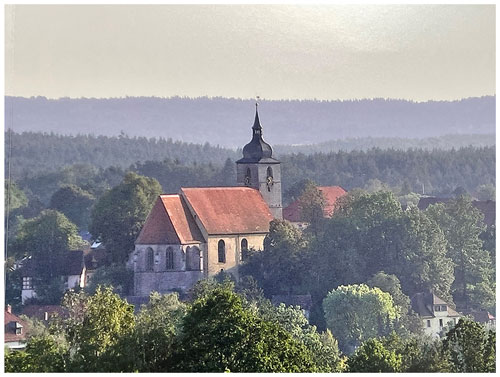
Figure 1Saint James Church (St. Jakobus-Kirche) in the old town of Creussen. The oldest altar part of the church is dated to 1477. It has appeared in its present form since 1700.
The excavations conducted by ReVe Büro für Archäologie GbR recovered human remains and artefacts from burials dated to as far back as the 9th century (personal communication with Claus Vetterling, chief of the ReVe Büro), thus providing a more secure estimate for the age of the Creussen settlement, which was first mentioned in written historic chronicles in 1003 (Kröll, 2003). The analysis of skeletal remains by advanced modern methods contributes to historical anthropology, palaeopathology, and many other archaeological disciplines seeking better understanding of prehistoric human health and diseases, as well as moving from bones to social behaviour (Katzenberg and Grauer, 2019). Examining material remains enables patterns of past human behaviour and cultural practices to be deduced.
During the previous excavations in the rear churchyard (der hintere Kirchhof at the northern side of Saint James Church) in 1989, about 20 burial places were recovered, which were dated to the 18th century (Kröll, 2003). In the dig along the northern wall of the church (Fig. 2), explored in August 2021, the archaeologists excavated dozens of burials and recovered numerous artefacts, such as ceramics, clothes fastening hooks, buckles, fragments of leather girdles, many sets of garment buttons, and others. Here we describe only 1 button from a set of 13 tin buttons (Fig. 3) of the same kind found in the burial located in the very eastern part of the dig, near the wall of the altar part of the church.
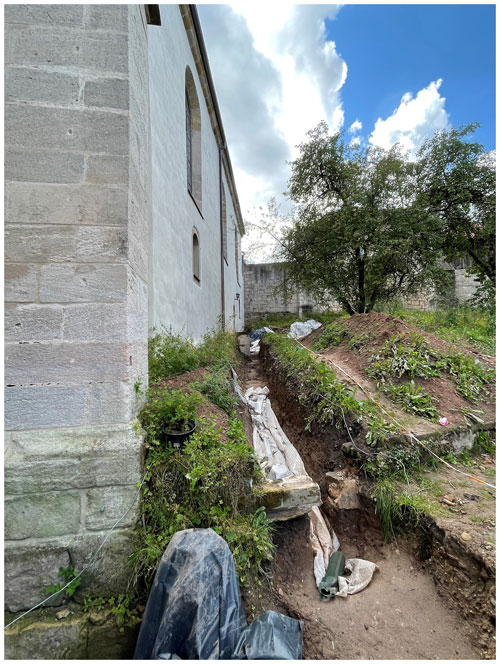
Figure 2The excavation site at the northern side of Saint James Church, where the tin buttons were found.
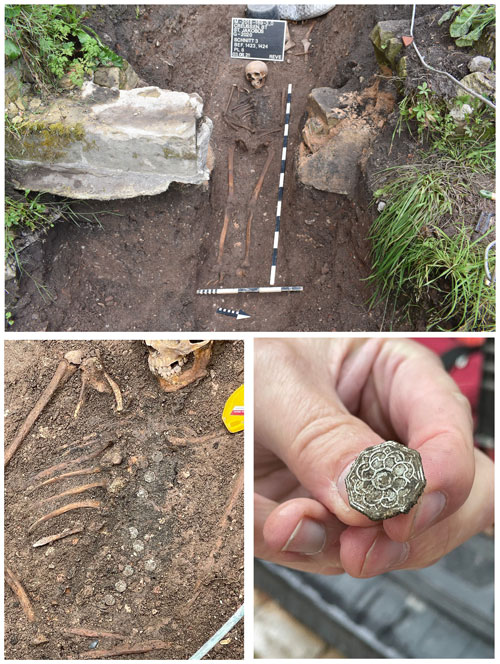
Figure 3The set of 13 tin buttons as found at the excavation site on the northern side of Saint James Church.
This is a one-piece cast button with a cast eye shank (Fig. 4). The face of the button has a floral decoration (Fig. 4a). The face and shank are cast as one unit, and the mold seam, well seen on the back (Fig. 4b, c), is passing through the shank also. Materials, basic construction techniques used for buttons manufacturing, and approximate dates for these techniques help archaeologists to more accurately assign termini post quem for buttons from archaeological deposits. According to the typology of 18th century metal buttons (Hughes and Lester, 1981, 1991; Hinks, 1988; Luscomb, 1967), the one we describe here can be regarded as type 1A2, metal waistcoat-size (19 mm) button, with the dates ranging from ca. 1720 to ca. 1800. Thus, 1720 can be assigned as the terminus post quem.
It is interesting to mention that visiting the Fränkische-Schweiz Museum in Pottenstein (Upper Franconia, Germany), the authors came across the information about men's clothes in the region in the 18th–19th centuries. Waistcoats worn at that time by men (Fig. 5) featured about a dozen buttons (Kretschmer, 1860/1870).
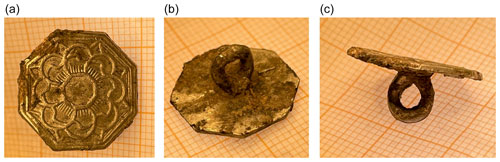
Figure 4Images of the button taken under an optical microscope. (a) The face of the button with a floral decoration. (b, c) The back of the button with the shank which reveals that this is a one-piece cast button with the eye separated from the back by a short stem: the face and shank are cast as one unit. A seam mold on the back is passing through the shank.
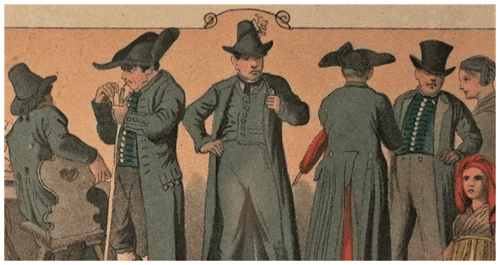
Figure 5Men's clothes in Upper Franconia in the 18th–19th centuries (Kretschmer, 1860/1870), courtesy of the Fränkische-Schweiz Museum. Waistcoats typically had about a dozen buttons.
The terminus ante quem (1796) could also be set due to the historical records and the church documents. According to Kröll (2003), the rear churchyard was in use until 1772 and closed in 1806; see Kröll (2003) and references therein. According to the four archival documents (files of the Creussen parish archive PfA Creußen 207, 566, 567, and 568; Fig. 6), which were examined on the authors' request (personal communication with Daniel Schönwald, State Church Archive of the Evangelical Lutheran Church in Bavaria – Landeskirchliches Archiv der Evangelisch-Lutherischen Kirche in Bayern (LAELKB)), considering also the church registers of the Evangelical Lutheran Creussen parish, the front and rear cemeteries were closed in 1796. Hence, the button recovered from the Saint James Church archaeological site in Creussen in 2021 could have been buried in the graveyard's soil for as long as 300 years. The material of the button and products of its weathering were studied by analytical methods.
The material of the button itself and products of its weathering found on the back of the button (Fig. 4b) were extracted and investigated using powder and single-crystal XRD, scanning electron microscopy, and energy-dispersive X-ray spectroscopy (SEM/EDX). SnO2 was used as a standard for quantitative EDX.
Powder and single-crystal XRD datasets were collected using a diffractometer equipped with a Bruker D8 platform (the 3-axis goniometer), an APEX detector, and an Ag Kα Incoatec I µS source (beam size of ∼ 50 µm FWHM (full width at half maximum)). The powder XRD data were collected in transmission geometry; the sample was rotated 360∘ during 60 to 300 s depending on the quality of material and sample size (ranging from 10 to 50 µm in linear dimensions). For studies of pre-selected single-crystal samples, we acquired half-sphere data with the exposure time of 60 s per frame with a step of 0.3∘ and 1265 frames in total. Lorentz and polarization corrections, as well as an analytical absorption correction based on the crystal shape, were taken into account for the correction of the reflection intensities using the CrysAlis package (CrysAlisPro Software System, 2019). All crystallographic data refinements were performed based on F2 using the SHELX97 programme package (Sheldrick, 2015) in the WinGX System (Farrugia, 1999). The chemical composition of the button was studied using scanning electron microscopy (ZEISS SEM, Leo Gemini 1530 with a Schottky field emission gun employing an accelerating voltage of 15–20 kV).
Two flakes of an approximate size of mm3 were cut with a scalpel from a metallic part of the button and another one (∼ mm3) from an interface between the metallic and weathered parts of this artefact. Powder X-ray diffraction of the metallic flakes corresponds to pure β-Sn (a tetragonal unit cell, lattice parameters a=5.831(2) and c=3.181(1) Å). SEM/EDX analysis confirms that the samples are pure tin. In the diffraction pattern of the flake taken from the interface, there are lines of other phases, apart from those of tin, which could be identified as cassiterite (SnO2) and romarchite (SnO). However, their lattice parameters were difficult to determine because their diffraction lines are broad and of low intensity. SEM/EDX analysis of the sample imaged in Fig. 7 shows the presence of tin oxides.
About 20 isometric particles of black or dark-yellowish colour of 20 to 30 µm in diameter were picked with a tungsten needle from the weathered part of the button (Fig. 8). Their examination using XRD shows the presence of continues Debye rings. Diffraction patterns of a few particles (richer in yellowish material) contain numerous spots apart from diffraction rings. Powder materials are cassiterite, SnO2 (tetragonal, typical lattice parameters a=4.735(1), c=3.184(1) Å), and romarchite, SnO (tetragonal, lattice parameters in different samples vary within 0.01 Å with typical values of a=3.801(2) and c=4.835(2) Å). SEM/EDX analysis confirms the presence of tin oxides in a very fine intergrowth (so it is impossible to characterize composition of individual minerals).
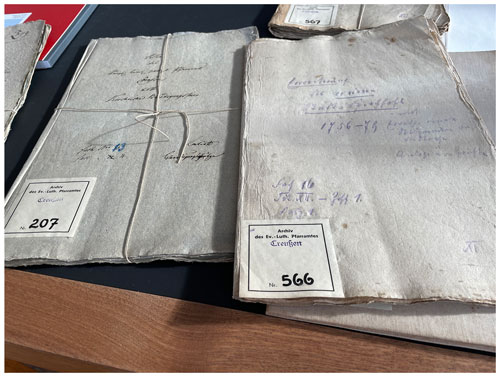
Figure 6A photograph of some of the documents from the State Church Archive of the Evangelical Lutheran Church in Bavaria (Landeskirchliches Archiv der Evangelisch-Lutherischen Kirche in Bayern (LAELKB)) inspected to establish terminus ante quem.
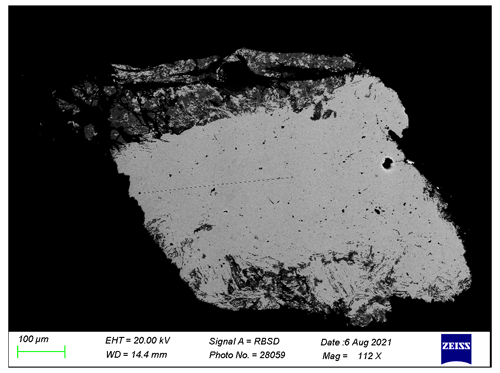
Figure 7SEM image in back-scattered electrons of a sample taken from the interface between the metallic and the weathered parts of the button. The gray area is the tin metal, and the black area corresponds to tin oxides.
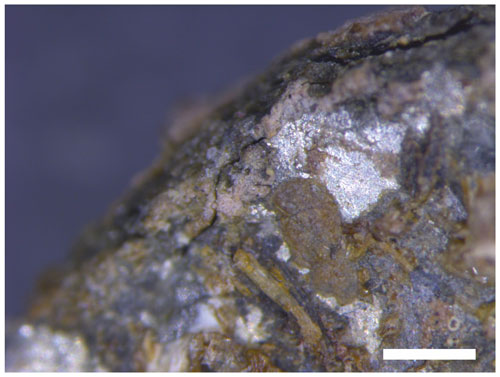
Figure 8Optical microscope image of the weathered part of the artefact. The shiny silver colour areas are tin; the black or dark-yellowish colour areas are weathered material. The scale (white bar) is 500 µm.
Four single-crystal datasets were collected for particles giving a spotty diffraction pattern. Apart from a cassiterite powder, these samples contain a tetragonal phase with unit cell parameters (a=7.9173(11) and c=9.0860(14) Å) that are in good agreement with those previously reported for hydroromarchite, Sn3O4H2, from SCXRD (a=7.93(1) and c=9.13(1) Å from Howie and Moser, 1968; a=7.8809(2) and c=9.0595(4) Å from Reuter and Schröder, 2010) and powder XRD (a=7.9268(4) and c=9.1025(5) Å) (Abrahams et al., 1996). Each particle contains dozens of randomly oriented domains of hydroromarchite that indicate that the size of individual crystals is just a few micrometres. The particle that gave the best SCXRD data was polished and investigated by SEM/EDX. SEM image of this sample in back-scattered electrons (Fig. 9) looks quite homogeneous with darker dots that may correspond to cassiterite. Chemical analysis (EDX) confirms that only two elements, tin and oxygen, are present in an approximate atomic proportion of 3:4.
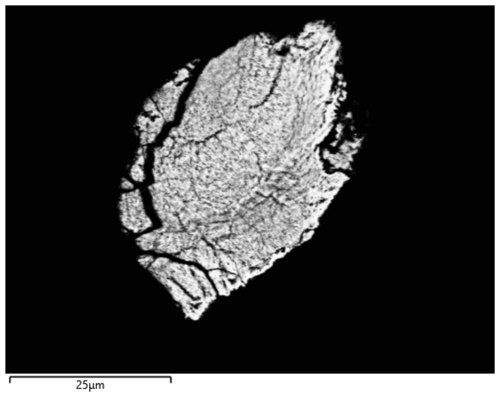
Figure 9SEM image in back-scattered electrons of a particle, which was studied by SCXRD. It contains dozens of randomly oriented domains of hydroromarchite and a powder of cassiterite.
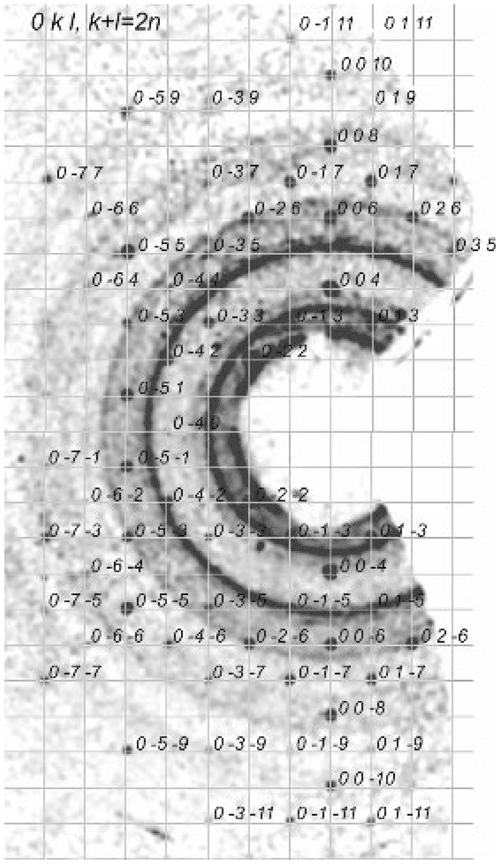
Figure 10Slice of the (0kl) reciprocal space of a hydroromarchite single crystal. Reflection condition corresponds to the space groups P4/mnc or P4nc. The systematic absences do not support the 21c space group, which was suggested by Ramik et al. (2003) and Abrahams et al. (1996) on the basis of their powder diffraction data. Powder lines are due to cassiterite.
Only one SCXRD dataset has sufficient quality for structure solution and refinement (Table 1). The quality of natural crystalline material is moderate (it scatters only to ∼ 0.9 Å) but satisfactory for reliable structural analysis. The structure of hydroromarchite has the space group P4/mnc(#128) with two Sn and one O atoms on crystallographically distinct positions (see Table 1 and the Crystallographic Information File (CIF) for the full crystallographic data). The space group P4/mnc was chosen between the two possible ones (P4/mnc or P4nc, based on systematic absences; Fig. 10) because the structure refinement in the lower-symmetry group does not improve the R-factor. The space group determined in our study agrees with that reported by Howie and Moser (1968, 1973), also based on SCXRD at room temperature. The systematic absences (Fig. 10) do not support the lower-symmetry 21c (#114) space group, which was suggested by Abrahams et al. (1996) on the basis of their powder diffraction data and the Rietveld refinement of the structure of tin (II) oxyhydroxide. Abrahams et al. (1996) used “a starting model based on the isostructural lead compound in space group 21c”; thus the space group was chosen a priori. The same 21c space group was reported by Reuter and Schröder (2010) based on low-temperature SCXRD. Considering group–subgroup relations, we cannot exclude that the lower symmetry found by Reuter and Schröder (2010) is due to the low-temperature conditions of their SCXRD measurements. However, in the absence of the structure refinement details in the publication by Reuter and Schröder (2010) – temperature, atomic coordinates, and atomic displacement parameters to compare with – we would rather refrain from any further speculations concerning the discrepancy.
The structural unit of Sn3O4H2 is a Sn6O8 cluster (Fig. 11a) consisting of oxygen atoms in the corners of an approximately regular cube (the cube's edge length of 2.56 Å vs. 2.82 Å in Howie and Moser, 1968) superimposed on an octahedral cluster of tin atoms (the octahedron's edge length of 3.55 Å vs. 3.59 Å in Howie and Moser, 1968). An average Sn–O distance (2.22 Å) in hydroromarchite is the same as in romarchite (Izumi, 1981), supporting the Sn(II) oxidation state of tin. Assuming Sn(II), the bond valence sum on oxygen atoms is equal to 1.5; then one H+ linked to two oxygen atoms provides the balance of valences (note that for Sn(III) or Sn(IV) a formal balance is not possible). Using the bond valence parameters from the dataset deposited at the IUCr web page (IUCr resources, 2020) and considering hydrogen to be at the middle between two oxygen atoms, one gets the bond valence sum on oxygen equal to 1.89 (with the same parameters the corresponding sum for romarchite is equal to 1.88). Layers of Sn6O8 clusters, oriented differently in adjacent layers, alternate in the c direction (Fig. 11b). Centres of the clusters form a body-centred tetragonal bct lattice. The distance between oxygen atoms of adjacent Sn6O8 clusters in successive layers, connected by hydrogen bonds, is 2.80 Å vs. 2.60 Å in Howie and Moser (1968) and is considerably less than the oxygen–oxygen distance between clusters in the same layer (4.62(5) vs. 4.60 Å in Howie and Moser, 1968). It should be pointed out that the length of the shortest inter-cluster contacts (2.60 Å) in Howie and Moser (1968) was found to be significantly shorter than the oxygen–oxygen distance within a single cluster (2.82 Å), which is not logical from a crystal–chemical point of view. In our model the inter-cluster O–O distance is equal to 2.56 Å and can become even less (down to ∼ 2.3 Å) in metal–organic analogues of a tin hydroxide (Suslova et al., 2007). The precise position of the hydrogen atoms, “symmetrically or statistically placed between oxygen atoms”, was not discussed in Howie and Moser (1968) but advised to be studied. Our investigation suggests a statistical distribution of hydrogen atoms in the structure of hydroromarchite, as we see that the Sn6O8 cluster is not distorted. In contrast, an ordered distribution of ligands in metal–organic analogues leads to considerable distortion of the Sn6O8 clusters (Suslova et al., 2007).
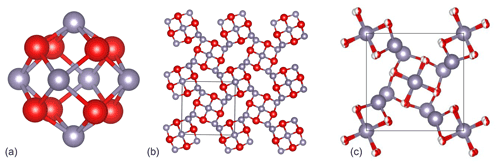
Figure 11The crystal structure of hydroromarchite. (a) A Sn6O8 cluster representing a structural unit and consisting of oxygen atoms (red balls) in the corners of an approximately regular cube superimposed on an octahedral cluster of tin atoms (grey balls); c axis is vertical in the figure. (b) Ball-and-stick model of the Sn3O4H2 structure viewed along the c direction. Centres of the Sn6O8 clusters form a body-centred tetragonal bct lattice. This model corresponds to the one proposed by Howie and Moser (1968) with a single O1 position. (c) Ball-and-stick model in which O1 position is split into two, O1′ and O1′′, with the occupancy equal to 0.5 (see Table 1). The splitting leads to the improvement of the thermal parameter of oxygen (Uiso ∼ 0.04 Å2), thus suggesting that this model correctly represents the structure of Sn3O2(OH)2.
Note that the structural model of hydroromarchite proposed by Howie and Moser (1968) and described above contains only one type of oxygen (i.e. all O atoms are in the same crystallographic position), and two hydrogen atoms per formula unit have to be added for a charge balance. Thus, in this structural model the formula Sn3O4H2 would have to be chosen because a choice of Sn3O2(OH)2 would mean two types of oxygen in the crystal structure. Both formulas were, however, suggested by Howie and Moser (1968) to interpret the analytical formula of hydroromarchite, probably based on their chemical intuition. If we consider the shortest contacts between clusters we found, they are ∼ 2.80 Å, which is too long for a symmetric hydrogen bond. As currently understood, in hydrous compounds with a common oxygen–hydrogen–oxygen sequence, a hydrogen bond is asymmetric (O–H⋯O) due to thermally activated hydrogen mobility at low compression, which often symmetrises (O–H–O) under increasing pressure (Meier et al., 2022). This hydrogen mobility causes an effective displacement of O atoms involved in the hydrogen bond. Indeed, the thermal ellipsoid of the oxygen atom is elongated, and in isotropic approximation it is quite large (Uiso ∼ 0.08 Å2; Table 1). Splitting the O1 position into two, O1′ and O1′′ with the occupancy equal to 0.5 (Table 1), leads to the improvement of the thermal parameter of oxygen (Uiso ∼ 0.04 Å2), and thus the structure of Sn3O2(OH)2 should be correctly presented, as shown in Fig. 11c.
In this work we report a new locality for the rare mineral hydroromarchite, Sn3O2(OH)2. It was found not in a submarine environment but in soil, at the Saint James Church archaeological site in Creussen, Germany. A tin artefact (a tin button) was exposed to weathering in soil for about 300 years. The mineral was identified using single-crystal X-ray diffraction. Its structure was solved and refined with the R-factor of 6.9 % providing more accurate crystallographic data than currently available for hydroromarchite.
All data derived from this research are available upon request from the corresponding author.
ND initiated the project. ND and MM selected the artefact. ND and LD performed the data collection and evaluation. MM oversaw archaeological interpretation. All authors discussed the results. ND wrote the paper with input from LD and MM.
The contact author has declared that none of the authors has any competing interests.
Publisher’s note: Copernicus Publications remains neutral with regard to jurisdictional claims in published maps and institutional affiliations.
We thank the following persons who kindly supported this study: Claus Vetterling (ReVe Büro für Archäologie GbR, Bamberg) for granting Natalia Dubrovinskaia and Leonid Dubrovinsky admission to the archaeological site in Creussen and his team, especially Verena Stürner, for sharing their knowledge in history and archaeology during the excavation time in Creussen in August 2021; Achim Peter (pastor of Saint James Church, Creussen) for helpful communications; Daniel Schönwald (Landeskirchliches Archiv der Evangelisch-Lutherischen Kirche in Bayern, LAELKB, Nürnberg) for his help in searching for documents in the church archive; Fabian Wittenborn and Jens Kraus (Fränkische-Schweiz Museum, Pottenstein) for their help in ethnographic questions; Alexander Wölfel (Schnabelweid) and Gernot Gebauer (Creussen) for sharing with the authors their collections of ancient buttons found in the fields around Creussen in Upper Franconia; and Dorothea Wiesner (University of Bayreuth), who helped in SEM analysis. Natalia Dubrovinskaia thanks Vadim Kessler and the Department of Molecular Sciences (Swedish University of Agricultural Sciences (SLU), Uppsala, Sweden) for involving her in the August T. Larsson Guest Researcher Program. Natalia Dubrovinskaia is also grateful to Vadim Kessler and Gulaim Seisenbaeva for sparking her interest in weathering processes, for fruitful discussions during the preparation of her lectures on mineral weathering in the course on environmental geochemistry taught at the SLU in the winter semester of 2021, and for helpful references concerning crystal chemistry of metal–organic analogues of a tin hydroxide.
This open-access publication was funded by the University of Bayreuth.
This paper was edited by Cristian Biagioni and reviewed by Andrew Locock and two anonymous referees.
Abrahams, I., Grimes, S. M., Johston, S. R., and Knowles, J. C.: Tin(II) oxyhydroxide by X-ray powder diffraction, Acta Crystallogr. C, 52, 286–288, 1996.
Berger, D., Soles, J. S., Giumlia-Mair, A. R., Brügmann, G., Galili, E., Lockhoff, N., and Pernicka, E.: Isotope systematics and chemical composition of tin ingots from Mochlos (Crete) and other Late Bronze Age sites in the eastern Mediterranean Sea: An ultimate key to tin provenance?, PloS ONE, 14, e0218326, https://doi.org/10.1371/journal.pone.0218326, 2019.
CrysAlisPro Software System: Version 1.171.40.84a, Rigaku Oxford Diffraction, Oxford, UK, 2019.
Di Martino, D., Cippo, E. P., Kockelmann, W., Scherillo, A., Minniti, T., Lorenzi, R., Malagodi, M., Merlo, C., Rovetta, T., Fichera, G. V., and Albano, M.: A multidisciplinary non-destructive study of historical pipe organ fragments, Mater. Charact., 148, 317–322, 2019.
Donaldson, J. D.: Crystalline hydrous tin (II) oxide, Acta Crystallogr., 14, p. 65, https://doi.org/10.1107/S0365110X61000164, 1961.
Dunkle, S. E., Craig, J. R., Rimstidt, J. D., and Lusardi, W. R.: Romarchite, hydroromarchite and abhurite formed during the corrosion of pewter artifacts from the Queen Anne's Revenge (1718), Can. Mineral., 41, 659–669, 2003.
Dunkle, S. E., Craig, J. R., and Lusardi, W. R.: Romarchite and associated phases as common corrosion products on pewter artifacts from marine archaeological sites, Geoarchaeology, 19, 531–552, 2004.
Farrugia, L. J.: WinGX suite for small-molecule single-crystal crystallography, J. Appl. Cryst., 32, 837–838, 1999.
Garuti, G. and Zaccarini, F.: Minerals of Au, Ag, and U in volcanic-rock associated massive sulfide deposits of the Northern Apennine ophiolite, Italy, Can. Mineral, 43, 935–950, 2005.
Hazen, R. M., Grew, E. S., Origlieri, M. J., and Downs, R. T.: On the mineralogy of the “Anthropocene Epoch”, Am. Mineral., 102, 595–611, 2017.
Hill, R. J.: Structure of Pb3O2(OH)2 by Rietveld analysis of neutron powder diffraction data, Acta Cryst. C, 41, 998–1003, 1985.
Hinks, S.: A Structural and Functional Analysis of Eighteenth Century Buttons, Dissertations, Theses, and Masters Projects, William & Mary, Paper 1539625441, https://doi.org/10.21220/s2-p512-7653, 1988.
Howie, R. A. and Moser, W.: Structure of tin (II) “hydroxide” and lead (II) “hydroxide”, Nature, 219, 372–373, 1968.
Howie, R. A. and Moser, W.: Crystal data and formula for hydrous tin(ll) oxide, Am. Min., 58, 552 pp., 1973.
Hughes, E. and Lester, M.: The big book of buttons, Boyerstown Pa, Boyerstown Publishing Company, ISBN: 0962904600, 1981.
Hughes, E. and Lester, M.: The book of buttons, New Leaf Publishers, ISBN 13: 9780962904608, 1991.
IUCr resources: Bond valence parameters, bvparm2020.cif, https://www.iucr.org/resources/data/datasets/bond-valence-parameters (last access: 9 January 2020), 2020.
Izumi, F: Pattern-fitting structure refinement of tin(II) oxide, J. Solid State Chem., 38, 381–385, 1981.
Katzenberg, M. A. and Grauer, A. L.: Biological Anthropology of the Human Skeleton, Wiley Blackwell, ISBN-10: 1119151619, ISBN-13: 978-1119151616, 2019.
Kretschmer, A.: Album Deutscher Volkstrachten. Original-Zeichnungen mit erklärenden Notizen, Leipzig, J. G. Bach's Verlag ohne Jahr, 1860/1870.
Kröll, J.: Geschichte von Creussen. Verlag Sendner & Neubauer, Bad Neustadt/Creuusen, ISBN: 3-8330-0393-6, 2003.
Luscomb, S. C.: The collector's encyclopedia of buttons, Crown Publischers Inc., New York, ISBN-10: 0764308890, ISBN-13: 978-0764308895, 1967.
Meier, T., Trybel, F., Khandarkhaeva, S., Laniel, D., Ishii, T., Aslandukova, A., Dubrovinskaia, N., and Dubrovinsky, L.: Structural independence of hydrogen-bond symmetrisation dynamics at extreme pressure conditions, Nat. Commun., 13, 3042, https://doi.org/10.1038/s41467-022-30662-4, 2022.
Nickel, E. H.: Definition of a mineral, Can. Mineral., 33, 689–690, 1995.
Nickel, E. H. and Grice, J. D.: The IMA Commission on New Minerals and Mineral Names: Procedures and guidelines on mineral nomenclature, Can. Mineral., 36, 17–18, 1998.
Organ, R. M. and Mandarino, J. A.: Romarchite and hydroromarchite, two new stannous minerals, Can. Mineral., 10, 916, 1971.
Reuter, H. and Schröder, D.: Low-temperature single-crystal X-ray data of synthetic hydroromarchite, Sn6O4(OH)4, Acta Crystallogr. A, 66, s190–s191, 2010.
Ramik, R. A., Organ, R. M., and Mandarino, J. A.: On type romarchite and hydroromarchite from Boundary Falls, Ontario, and notes on other occurrences, Can. Mineral., 41, 649–657, 2003.
Sheldrick, G. M.: Crystal Structure Refinement with SHELXL, Acta Crystallogr. C, 71, 3–8, 2015.
Suslova, E. V., Turova, N. Y., Kessler, V. G., and Belokon', A. I.: Electrosynthesis of Tin(II) Alkoxides, Russ. J. Inorg. Chem., 52, 1682–1686, 2007.
- Abstract
- Introduction
- Hydroromarchite: an anthropogenic mineral originating from tin weathering
- New locality for anthropogenic hydroromarchite: the description of the Saint James Church (St. Jakobus-Kirche) archaeological site in Creussen, Germany, and the tin artefact
- Materials and methods
- Results and discussion
- Conclusions
- Data availability
- Author contributions
- Competing interests
- Disclaimer
- Acknowledgements
- Financial support
- Review statement
- References
- Abstract
- Introduction
- Hydroromarchite: an anthropogenic mineral originating from tin weathering
- New locality for anthropogenic hydroromarchite: the description of the Saint James Church (St. Jakobus-Kirche) archaeological site in Creussen, Germany, and the tin artefact
- Materials and methods
- Results and discussion
- Conclusions
- Data availability
- Author contributions
- Competing interests
- Disclaimer
- Acknowledgements
- Financial support
- Review statement
- References