the Creative Commons Attribution 4.0 License.
the Creative Commons Attribution 4.0 License.
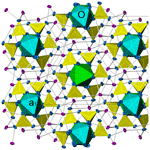
Heimaeyite, Na3Al(SO4)3, a new mineral from the fumaroles on Eldfell volcano, Iceland
Anna Garavelli
Donatella Mitolo
Fabrizio Nestola
Martha Giovanna Pamato
Maja Bar Rasmussen
Morten Bjerkvig Jølnæs
The new mineral, heimaeyite, was found among the fumarolic encrustations collected on Eldfell volcano, on the island of Heimaey, Iceland. The mineral formed as microscopic rounded aggregates (<50 µm) and rounded grains (<10 µm) mixed with tiny needles of koryakite and a mineral phase with an NaMgAl(SO4)3 composition. It was formed in scoria consisting of anhydrite, hematite, and cristobalite. Other associated minerals are tamarugite, hexahydrite, löweite, and langbeinite. Heimaeyite is orange with a yellowish streak. It is translucent with a vitreous lustre. The calculated density is 2.783 g cm−3. The chemical analysis gives a composition of Na2.93Al0.82Fe0.25S2.99O12.05. The ideal formula is Na3Al(SO4)3 with 10 % to 25 % replacement of Al with Fe. Single-crystal X-ray diffraction analysis shows that it is trigonal and has a space group of with unit cell parameters of a=13.4326(9) Å, c=8.9818(7) Å, V=1403.5(2) Å3, and Z=6. The X-ray powder diffraction resembles that of Na3V(SO4)3 (PDF 39-0243), with which it is isostructural. They both belong to the K7Nb(SO4)6 structure type. In the crystal structure, the octahedral coordinations of the cation (in heimaeyite this is Al) are connected by sulfate tetrahedra in a “pin-wheel” arrangement into chains extending along [001]. The chains are interconnected by [NaO6] distorted trigonal prism coordinations. Fe substitutes for Al in the larger of the two symmetry-independent octahedral sites. Synthetic pure Na3Al(SO4)3 was prepared through a solid-state reaction at 550 °C, and its structure was refined by the Rietveld method. It shows a smaller difference in size between the two octahedral sites than the natural sample.
- Article
(6580 KB) - Full-text XML
-
Supplement
(168 KB) - BibTeX
- EndNote
Jakobsson et al. (2008) published an overview of the mineralogy of fumaroles from three Icelandic volcanoes, where the samples were collected shortly after their eruptions (Surtsey in 1963–1967, Eldfell in 1973, and Hekla in 1991). They recorded a number of known fumarolic minerals plus some supposedly new minerals by powder X-ray diffraction (PXRD), which were given different two-letter labels. In 2009, the Eldfell volcano was visited by a team of geologists from the University of Copenhagen, led by the late Sveinn Jakobsson from the Icelandic Institute of Natural History. Among the samples collected then was the one that contained the mineral with PXRD characteristics similar to that observed in previous work and labelled “EN”. We have made a detailed investigation of the mineral, confirmed its novelty, and called it heimaeyite after the island of Heimaey (hosting the largest Icelandic fishing harbour of the same name) on which Eldfell volcano is situated (63°25′50′′ N, 20°14′48′′ W). The new mineral and its name were accepted by the Commission on New Minerals, Nomenclature and Classification (CNMNC) of the International Mineralogical Association (Bosi et al., 2024).
The holotype is kept in the mineral collection of the Icelandic Institute of Natural History in Garðabær, Iceland, under sample number NI-30218.
Heimaeyite formed in a tube inside red scoria consisting mostly of a mixture of anhydrite, hematite, and cristobalite through which fumarolic gases percolated. It formed a ceramic-like white-yellow-reddish mass that consists of an intimate mixture of heimaeyite; koryakite ((Na,K)MgAl(SO4)3); the mineral labelled “EA” that is still not fully described (NaMgAl(SO4)3); and products of their hydration: tamarugite, hexahydrite, and löweite plus some langbeinite. The measured temperature in the tube where the sample was collected was between 200 and 300 °C.
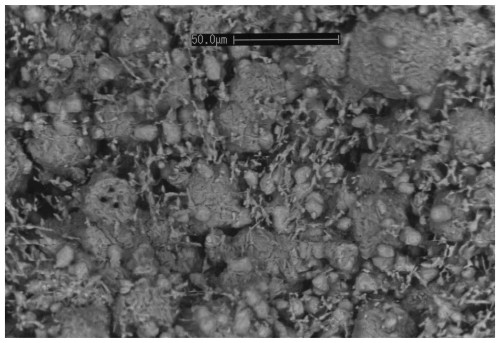
Figure 2SEM photograph of the aggregate of heimaeyite (rounded grains and globular aggregates) and the other new mineral EA plus koryakite (both acicular).
A scanning electron microscopy (SEM) investigation of the part of the sample containing heimaeyite showed that it was porous on the micrometre scale (Fig. 2). A measurement by an attached energy-dispersive spectrometer (EDS) helped to identify the globular aggregates of platy crystals and individual isometric crystals as heimaeyite and acicular crystals dispersed between them as EA or koryakite.
The colour of heimaeyite is orange (seen on the small fragment used for the single-crystal XRD analysis), the lustre is vitreous, and it is translucent. The streak is yellow. These observations apply for the natural sample that contained some Fe replacing Al. Pure synthetic Na3Al(SO4)3 is colourless, and its streak is white. Due to admixture with other minerals and small dimensions of the grains, fluorescence, hardness, and tenacity could not be investigated.
The cleavage was not observed and is not expected as no preferred orientation could be registered on PXRD diagrams. All observed fragments had uneven fractures.
Density could not be measured due to the characteristics of the sample. The density calculated on the basis of chemical analysis and the dimensions of the unit cell from single-crystal XRD is 2.783 g cm−3, and, calculated from the results of the crystal structure analysis, it is 2.747 g cm−3.
Magnetism was not observed.
Optical properties were not measured due to the size of the grains. The largest grain we could find (longest diameter was around 70 µm) was used for the XRD crystal structure analysis, and others were significantly smaller. The synthetic material had similar characteristics. The calculated mean refractive index, using the Gladstone–Dale relationship (Mandarino, 1976), is 1.5222.
A preliminary chemical analysis was done by an S 360 Cambridge SEM coupled with an Oxford Link Ge ISIS EDS, equipped with a Super Atmosphere Thin Window. It showed that heimaeyite consists of Na, Al, S, and O, giving an approximate formula of Na3Al(SO4)3, with a small additional amount of Fe. Koryakite and EA were also observed as associated minerals (Fig. 2).
A fragment of the sample was embedded in epoxy, polished with diamond powder in oil, and sputtered with a 30 nm thick carbon film prior to analysis by an electron microprobe analyser (EMPA; JEOL JXA-8200). The beam voltage and current were 15 kV and 5 nA, and the spot size was a 10 µm measurement time of 10 s, with 5 s measurement on each side of the maximum for subtraction of background being used. The 11 analyses were chosen with the smallest contribution of impurities, and their averages are reported in Table 1.
The low totals we explain by a high porosity of the sample, which adds to all the analysis measurements of some epoxy and embedded polishing diamond grains. We assume the presence of small amounts of Mn, Si, K, and Mg is due to impurities, with the latter two being contributed by the unavoidable small needles of the accompanying minerals (see Fig. 2).
The total composition of the sample results in K0.10Na2.95Mn0.03Mg0.12Fe0.25Al0.94Si0.01S3.35O13.5. If the Mn and Si impurity and the contributions of koryakite (NaKMg2Al2(SO4)6) and EA (NaMgAl(SO4)3) are removed, calculating the additional subtracted amounts of Al, Na, and S, which they also contain, with Al' equalling Mg, Na' equalling Mg–K, and S' equalling 3× Mg, the resulting empirical formula of heimaeyite is Na2.93Al0.82Fe0.25S2.99O12.05.
The ideal formula for the pure Al compound without Fe is Na3AlS3O12, which requires 24.20 wt % Na2O, 13.27 wt % Al2O3, and 62.53 wt % SO3.
Heimaeyite is soluble in water.
Table 2X-ray powder diffraction data (d in Å) for heimaeyite. * The intensities of the eight strongest maxima are in bold.
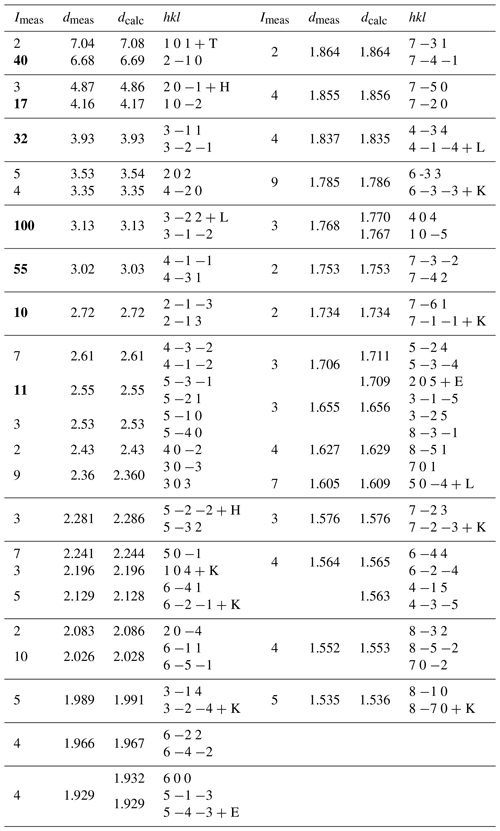
* Other minerals present in the sample: tamarugite (T), hexahydrite (H), langbeinite (L), koryakite (K), and the new mineral EA (E).
4.1 PXRD
PXRD data (Table 2) were collected with a Bruker AXS D8 ADVANCE powder diffractometer, operating in a Bragg–Brentano reflection geometry and equipped with a primary Ge111 monochromator and silicon-strip LYNXEYE detector. The radiation wavelength was CuKα1 (1.54059 Å), and the step scan was 0.02° with a measuring time of 4 s per step and a rotating sample holder. The PXRD diagram of a sample containing 41 wt % heimaeyite, 18 wt % tamarugite, 15 wt % koryakite, 11 wt % langbeinite, 9 wt % EA, and 7 wt % hexahydrite is represented in Fig. 3. Unit cell parameters refined from the powder data are as follows: a=13.3872(4) Å, c=8.9411(4) Å, V=1387.7(1) Å3. The crystal system is trigonal.
Because of the similarity of the XRD pattern to Na3V(SO4)3 (PDF 39-0243) and its association with eldfellite (NaFe(SO4)2), the mineral EN was previously considered to be isostructural with the former one but with a composition of Na3Fe(SO4)3 (Jakobsson et al., 2008). Only after the SEM–EDS measurement of the sample collected in 2009 was the compound revealed to be a predominantly aluminous isostructural compound. This was further confirmed by the single-crystal XRD study.
4.2 Single-crystal XRD
Single-crystal XRD studies were carried out with a Supernova diffractometer (Rigaku Oxford Diffraction) equipped with a micro (Mo) X-ray source (wavelength of 0.71073 Å) and a PILATUS DECTRIS 200K area detector. The crystal structure was solved and refined using the Jana2006 software (Petřiček et al., 2014).
The crystal structure was determined using the SUPERFLIP programme (Palatinus and Chapuis, 2007) incorporated into Jana, which uses the charge-flipping method (Oszlányi and Sütõ, 2004). The programme revealed the positions of all the atoms in the structure, which were then refined with Jana's refinement routine, finishing with the anisotropic refinement of atom displacement parameters (ADPs) for all atomic sites and the occupancies of Al and Fe at the Al2 site with their sum constrained to 1 and having the same ADP. The main details of the measurement and final refinement results are presented in Table 3, and Table 4 gives the atomic parameters. All details can be seen in the deposited Crystallographic Information File (CIF).
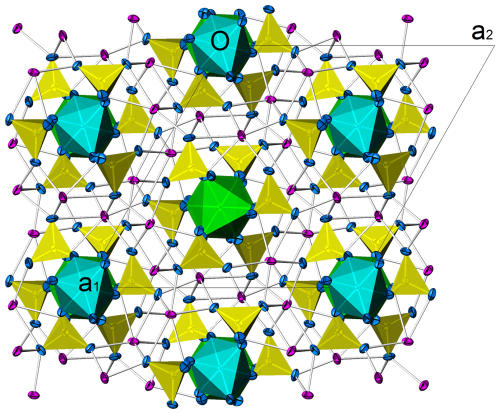
Figure 4The crystal structure of heimaeyite as seen along [001]. S coordinations are depicted as yellow tetrahedra; Al1 and Al2 coordinations are cyan and green octahedra, respectively; and Na and oxygen atoms are magenta and blue, respectively. Atomic displacement ellipsoids drawn at 50 % probability level. Drawn by Atoms (https://www.shapesoftware.com, last access: 1 October 2024).
5.1 Description of the structure
Heimaeyite is isostructural to Na3Fe(SO4)3 (Scordari et al., 2011) and Na3V(SO4)3 (Boghosian et al., 1994). They belong to the K7Nb(SO4)6–K7Ta(SO4)6 structure type (Borup et al., 1990). Heimaeyite's crystal structure can be seen in Fig. 4. It consists of the chains parallel to [001] formed by the [AlO6] coordination octahedra interconnected by [SO4] groups (neighbouring octahedra are connected by three sulfate groups). The chains form a 3D structure through a linkage to Na atoms that interconnect triplets of chains and which are in a distorted 6-fold coordination. The successive Al coordination octahedra in a chain are rotated relative to each other by approximately 180° around the c axis with a “pin-wheel” configuration of the octahedra and the attached sulfate groups. The c period encompasses two Al coordinations, and due to the space group symmetry, they are symmetrically independent. In heimaeyite, the [Al2O6] octahedron is significantly larger than [Al1O6]. We explain the difference by the incorporation of Fe into the larger octahedral site, which is confirmed by the crystal structure refinement of atomic occupancies (Table 4). Table 5 gives a list of bond lengths.
5.2 The relations between the structures with the K7Nb(SO4)6 structure type
There are five crystal structures with this structure type (Inorganic Crystal Structure Database, https://icsd.fiz-karlsruhe.de, last access: 1 October 2024, and this work) known so far, two with potassium as an alkali element, K7Nb(SO4)6 and K7Ta(SO4)6 (Borup et al., 1990), and three with sodium, Na3V(SO4)3 (Boghosian et al., 1994), Na3Fe(SO4)3 (Scordari et al., 2011), and Na3Al(SO4)3 (heimaeyite). There are two main differences between the potassium and the sodium structures. The first is that the potassic ones have two different atomic species with different valences at two independent octahedral sites, one of them being K, whereas the sodic ones have only one element type at both sites. The exception among the sodic ones is heimaeyite, which has partial occupancy of Fe on one of them (however, the pure Al compound can be synthesized as described later). The second difference is the coordination of the alkali site that connects tetrahedral–octahedral chains, which is a distorted octahedral site in the case of K (Fig. 5a) and a distorted trigonal prism in the case of Na (Fig. 5b).
Table 6The parameters of the coordination polyhedra in the K7Nb(SO4)6 structural group calculated with the IVTON programme (Balić Žunić and Vicković, 1996). str: structure (1: heimaeyite, Na3Al1−xFex(SO4)3; 2: Na3V(SO4)3; 3: K7Nb(SO4)6; 4: K7Ta(SO4)6); CN: coordination number; : average bond length; bvs: bond valence sum; Vp: polyhedral volume; vd: volume distortion; asp: asphericity; ecc: eccentricity. The data for Na3Fe(SO4)3 are not given because this structure has been determined and refined at a high temperature (390 °C) from a powder sample (Scordari et al., 2011), whereas the other structures were refined from single-crystal data collected at room temperature with significantly higher accuracy.
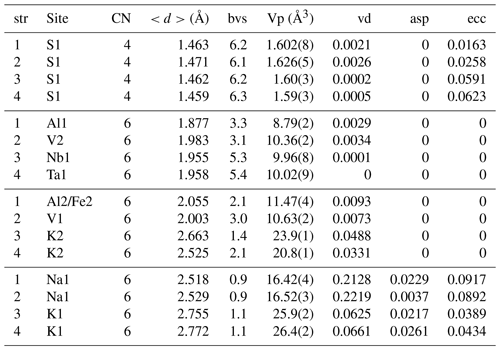
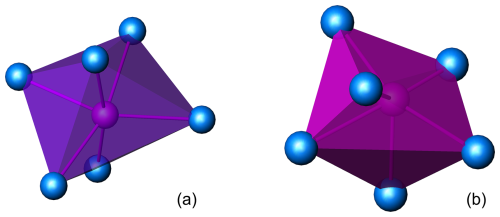
Figure 5(a) The coordination of K1 in the structure of K7Nb(SO4)6. The projection is on the (110) plane with [001] oriented vertically. (b) The coordination of Na1 in the structure of heimaeyite. The projection has the same orientation as in (a).
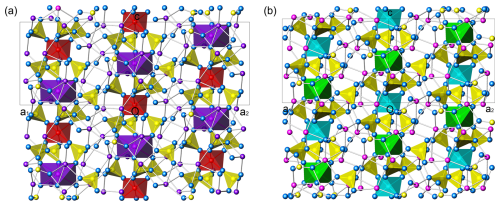
Figure 6(a) The (110) slice from the structure of K7Nb(SO4)6. Nb coordinations are red, S coordinations are yellow, K1 atoms and K2 coordinations are dark violet, and O atoms are blue. (b) The (110) slice from the structure of heimaeyite. The colours are like those in Fig. 4.
The values of volume distortions in Table 6 illustrate these characteristics. For Na1 in Na3V(SO4)3, there is a seventh O atom just closer than 3 Å and its coordination was reported as 7-fold (Boghosian et al., 1994), but here we included only the closest six oxygens in the calculations for the sake of comparison with the other structures. The “maximum-volume” polyhedron for coordination number 6 is the regular octahedron (volume distortion of 0). The volume distortion for the archimedean trigonal prism (all edges of equal length) calculated relative to the octahedron is 0.2711 (Makovicky and Balić-Žunić, 1998). The discrepancy of these values measures the amount of distortion from the ideal types. The values for both K1 and Na1 lie between the values for the octahedron and trigonal prism but are closer to the octahedron for K and to the trigonal prism for Na.
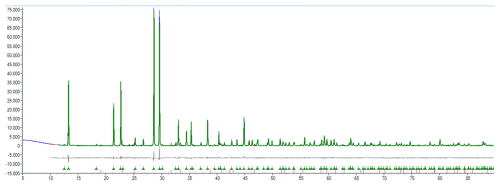
Figure 7PXRD pattern of the sample containing 95 wt % synthetic Na3Al(SO4)3 and 5 wt % synthetic metathénardite (Na2SO4) with the results of the Rietveld refinement. Blue: observed pattern, red: calculated pattern, green: calculated pattern of Na3Al(SO4)3, grey: difference observed − calculated, green ticks: positions of Na3Al(SO4)3 diffraction maxima, magenta ticks: positions of metathénardite diffraction maxima.
Table 7The crystal structure parameters of synthetic Na3Al(SO4)3 obtained by Rietveld refinement of powder XRD.
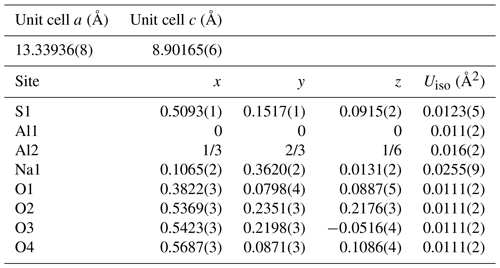
The characteristics of the crystal structure type explain the appearance of the differences between the potassium and sodium members. As can be seen in Table 6, the octahedral coordinations in chains differ in the degree of distortion. The only distortion in their case is the volume distortion; the eccentricity and asphericity are zero because the central cations sit on the symmetry centre of and all the bond lengths are equal. The discrepancy from an ideal octahedral coordination can be either the elongation or shortening along the 3-fold axis (it is the shortening in all of the aforementioned structures). The distortion is larger for the larger octahedron (corresponding to Al2/Fe2 in heimaeyite), especially in the cases when K occupies this site when the coordination becomes significantly flattened (see Fig. 6a). For comparison, Fig. 6b shows the structure of heimaeyite projected along the same crystallographic direction. As can be seen in Table 6, the bond valence sums for this site show large discrepancies as expected, except for Na3V(SO4)3, where it is occupied by the same species as the (slightly) smaller octahedron. Despite the discrepancy of bond valence sums for cations in the large chain octahedra, the bond valence sums for O atoms and other cations in all structures correspond well to the expectation, suggesting that the sizes of large octahedral coordinations do not come from mistakes in the structure refinement. The volume distortion of the sulfate tetrahedra is small in all structures (Table 6). The largest distortion in them is the eccentricity of the S atom, which has two shorter and two longer bonds. The latter are bonds to oxygens shared with the cations in the octahedral coordinations in chains. The quasi-rigidity of the sulfate tetrahedra, connecting the symmetry-constrained octahedral coordinations, allows for the adjustment of the size of octahedra by a rotation of tetrahedra so that a significantly larger octahedral cation in one site can be combined with the smaller ones in the neighbouring octahedral sites, like in the case of K+ with Nb5+ or Ta5+. This applies for the heimaeyite structure as well, where larger Fe3+ substitutes for Al3+ only at the Al2 site. The rotation of sulfate tetrahedra also influences the coordination of alkali cations that sit between the chains. In the case of drastic differences in the size of the chain octahedra, K atoms situated between chains find themselves in a coordination environment different from that of the Na atoms, which are situated between chains with minor differences in the sizes of chain octahedra.
The existence of isostructural Na3Al(SO4)3 and Na3Fe(SO4)3 and the detected moderate Fe for Al substitution in heimaeyite suggest the possibility of a solid solution between these two compounds. A description of a phase from the burnt mine dumps of the Chelyabinsk coal basin with a composition of (K,Na)3Na3(Fe,Al)2(SO4)6 (Zolotarev et al., 2020) may signify that an ideal solid solution cannot be achieved, in any case not in a complete form and not without structural complications. The crystal structure reported for the Chelyabinsk phase shows that it has the same space group () as heimaeyite but with an approximately doubled c period. It features the octahedral–tetrahedral chains with a similar topology, but they have three symmetry-independent, mixed Fe–Al octahedral sites, all dominated by Fe. The interconnection between the chains is different from heimaeyite, due to two symmetry-independent alkali sites, one of them is dominated by K with minor Na substitution and the other is purely Na. It is, therefore, presently not clear whether the structural differences result solely from K–Na substitution or also from Fe–Al mixing complexity.
The mineral labelled EN (Jakobsson et al., 2008) was found in a frothy crust, 2–3 cm thick, with various shades of brownish-to-greenish and whitish-yellow colour, covering altered scoria of hawaiite, in association with eldfellite, tamarugite, anhydrite, blödite, and hematite (Balić-Žunić et al., 2009). The crystal size and the admixture with other minerals prevented a further characterization of EN, apart from a PXRD diagram where it is one of the four phases. Whether it is close to heimaeyite in composition or closer to Na3Fe(SO4)3, as originally supposed because of the association with eldfellite (NaFe(SO4)2), remains an open question. The results from the Rietveld refinement of the original EN diagram (the accuracy is significantly lower than the present one of heimaeyite) give a value of the a unit cell parameter closer to that of Na3Al(SO4)3, whereas the c parameter is closer to that of Na3Fe(SO4)3. Therefore, they do not allow for a definite conclusion.
Synthetic Na3Al(SO4)3 was prepared by heating a mixture of powders of Na2SO4 and Al3(SO4)2(H2O)17 in stoichiometric proportions in a platinum crucible for 1 month at 550 °C. At the end, the sample contained Na3Al(SO4)3 with a small remaining amount of Na2SO4.
The product was then analysed at room temperature with the same instrument and conditions as used for the powder XRD analysis of heimaeyite. The Rietveld refinement was done starting with the crystal structure parameters of heimaeyite and the parameters for metathénardite from Eysel et al. (1985). For Na3Al(SO4)3 the occupancy of Al2 was constrained to 1 (Al), while the positional parameters, isotropic ADP (those of O atoms constrained to be equal), and unit cell parameters were refined. For metathénardite (amounting to 5 wt %) only the unit cell parameters were refined. The final reliability factors were Rwp=9.65 % and Rp=6.70 %. The RBragg for Na3Al(SO4)3 was 2.00 %.
The crystal structure parameters of synthetic Na3Al(SO4)3 are reported in Table 7, and the PXRD pattern is in Fig. 7.
As can be seen from Table 8, the main difference in the structure of synthetic Na3Al(SO4)3 to that of heimaeyite is in the coordination polyhedron of Al2 (now a pure Al site), which is not much larger than that of Al1, and there is the seventh O atom coming into the coordination sphere of Na1. The situation in synthetic, pure Al compound resembles the crystal structure of Na3V(SO4)3. However, the crystal structure of synthetic Na3Al(SO4)3 has been refined from PXRD data with a much smaller number of observed reflections and many constraints in ADP, so it is expected to be of lesser accuracy than the one obtained for heimaeyite from single-crystal XRD data.
Na3Al(SO4)3 was also previously prepared by dissolving stoichiometric quantities of Na sulfate and Al sulfate in water and then calcining the precipitate at 500 °C (Martínez-Lope et al., 1989) and by a reaction of kaolinite with NaHSO4 at high temperatures (Colina and Costa, 2005). To the best of our knowledge, the only other anhydrous Na–Al sulfate known is synthetic NaAl(SO4)2 (Boujelbene and Mhiri, 2013).
The new mineral from the fumaroles of Eldfell volcano on the Icelandic island of Heimaey was shown to be isostructural with Na3V(SO4)3 (Boghosian et al., 1994) and has a composition of Na3Al(SO4)3 with a substitution of 10 %–25 % Al by Fe. It was given the name heimaeyite after the island on which it was found.
Pure Na3Al(SO4)3 has also been synthesized at 550 °C.
Na3Al(SO4)3 crystallizes in the space group with a c:a ratio of 0.6687 and has a K7Nb(SO4)6 structure type similar to Na3V(SO4)3, Na3Fe(SO4)3, and K7Ta(SO4)6. In the crystal structure of heimaeyite, Al is in octahedral coordination with O atoms at two symmetry-independent sites, which are connected by sulfate tetrahedra in a pin-wheel configuration in chains along [001]. The chains are connected to a 3D structure by [NaO6] coordinations in the form of distorted trigonal prisms. Fe is substituted in minor quantities for Al in the larger of the two octahedral coordinations.
To understand the eventual solid solution of Al and Fe in this structure type, the mixing behaviour has still to be investigated along the join of Na3Al(SO4)3–Na3Fe(SO4)3.
Crystallographic data for heimaeyite are available in the Supplement.
The supplement related to this article is available online at https://doi.org/10.5194/ejm-37-79-2025-supplement.
TBZ performed the XRD measurements, led the investigation, and prepared the manuscript with contributions from all co-authors. AG and DM performed the SEM investigations. FN and MGP assisted with the single-crystal measurements. MBR performed EMPA measurements. MBJ contributed to the initial investigations.
The contact author has declared that none of the authors has any competing interests.
Publisher’s note: Copernicus Publications remains neutral with regard to jurisdictional claims made in the text, published maps, institutional affiliations, or any other geographical representation in this paper. While Copernicus Publications makes every effort to include appropriate place names, the final responsibility lies with the authors.
This article is part of the special issue “New minerals: EJM support”. It is not associated with a conference.
Tonči Balić-Žunić, Anna Garavelli, Donatella Mitolo, and Morten Bjerkvig Jølnæs gratefully remember the late Svein P. Jakobsson, who inspired the investigations of Eldfell fumaroles and also led the expedition in 2009. Tonči Balić-Žunić, Anna Garavelli, and Donatella Mitolo are also indebted to the late Filippo Vurro, who promoted the joint cooperation which led to the characterization of a number of new minerals among Icelandic fumarolic encrustations. We thank Ljiljana Karanović for a critical reading of the paper and comments, Stefanie Lode from the Norwegian University of Science and Technology and Janet Zulauf from Goethe University Frankfurt for the preparation of the sample for microprobe measurement, and Tod E. Waight for help in setting up the microprobe conditions. We also thank Anna Katerinopoulou and Dora Balić Žunić, who participated in the expedition and helped with the collection of samples.
This paper was edited by Sergey Krivovichev and reviewed by two anonymous referees.
Balić Žunić, T. and Vicković, I.: IVTON – Program for the Calculation of Geometrical Aspects of Crystal Structures and Some Crystal Chemical Applications, J. Appl. Crystallogr., 29, 305–306, 1996.
Balić-Žunić T., Garavelli A., Acquafredda P., Leonardsen E., and Jakobsson S. P.: Eldfellite, NaFe(SO4)2, a new fumarolic mineral from Eldfell volcano, Iceland, Mineral. Mag., 73, 51–57, https://doi.org/10.1180/minmag.2009.073.1.51, 2009.
Bosi, F., Hatert, F., Pasero, M., and Mills, S. J.: IMA Commission on New Minerals, Nomenclature and Classification (CNMNC) – Newsletter 81, Eur. J. Mineral., 36, 917–923, https://doi.org/10.5194/ejm-36-917-2024, 2024.
Boghosian, S., Fehrmann, R., and Nielsen, K.: Synthesis and crystal structure of Na3V(SO4)3. Spectroscopic characterisation of Na3V(SO4)3 and NaV(SO4)2, Acta Chem. Scand., 48, 724–731, 1994.
Borup, F., Berg, R. W., and Nielsen, K.: The crystal structure of K7Nb(SO4)6 and K7Ta(SO4)6, Acta Chem. Scand., 44, 328–331, 1990.
Boujelbene, M. and Mhiri, T.: Ab initio X-ray structure determination of NaAl(SO4)2, J. Adv. Chem., 5/1, 607–613, https://doi.org/10.24297/JAC.V5I1.941, 2013.
Colina, F. G. and Costa, J.: High-Temperature Reaction of Kaolin with Sodium Hydrogen Sulfate, Ind. Eng. Chem. Res., 44, 4495–4500, https://doi.org/10.1021/ie030273d, 2005.
Eysel, W., Höfer, H., Keester, K., and Hahn, T.: Crystal chemistry and structure of Na2SO4 (I) and its solid solutions, Acta Crystallogr., B41, 5–11, 1985.
Jakobsson, S. P., Leonardsen, E. S., Balic-Zunic, T., and Jonsson, S. S.: Encrustations from three recent volcanic eruptions in Iceland: The 1963–1967 Surtsey, the 1973 Eldfell and the 1991 Hekla eruptions, Fjölrit Náttúrufraedistofnunar, 52, 65 pp., ISSN 1027-832X, 2008.
Makovicky, E. and Balić-Žunić, T.: New measure of distortion for coordination polyhedra, Acta Crystallogr., B54, 766–773, 1998.
Mandarino, J. A.: The Gladstone-Dale relationship – Part I: Derivation of new constants, Can. Mineral., 14, 498–502, 1976.
Martínez-Lope, M., Zamora, J., and García-Clavel, M. E.: Oxysalt – metal double reactions in the solid state: Na3Al(SO4)3 – Mg, Thermochim. Acta, 153, 105–111, 1989.
Oszlányi, G. and Sütõ, A.: Ab initio structure solution by charge flipping, Acta Crystallogr., A60, 134–141, https://doi.org/10.1107/S0108767303027569, 2004.
Palatinus, L. and Chapuis, G.: SUPERFLIP – a computer program for the solution of crystal structures by charge flipping in arbitrary dimensions, J. Appl. Crystallogr., 40, 786–790, https://doi.org/10.1107/S0021889807029238, 2007.
Petřiček, V., Dušek, M., and Palatinus, L.: Crystallographic Computing System JANA2006: General Features, Z. Kristallogr., 229, 345–352, https://doi.org/10.1515/zkri-2014-1737, 2014.
Scordari, F., Ventruti, G., Gaultieri, A. F., and Lausi, A.: Crystal structure of Na3Fe(SO4)3: A high-temperature product (∼400 ° C) of sideronatrite [Na2Fe(SOH2O], Am. Mineral., 96, 1107–1111, https://doi.org/10.2138/am.2011.3783, 2011.
Zolotarev, A. A., Krivovichev, S. V., Avdontceva, M. S., Shilovskikh, V. V., Rassomakhin, M. A., Yapaskurt, V. O., and Pekov, I. V.: Crystal Chemistry of Alkali–Aluminum–Iron Sulfates from the Burnt Mine Dumps of the Chelyabinsk Coal Basin, South Urals, Russia, Crystals, 10, 1062, https://https://doi.org/10.3390/cryst10111062, 2020.
- Abstract
- Introduction
- Appearance and physical properties
- Chemical analysis
- X-ray diffraction analysis
- The crystal structure
- Synthetic Na3Al(SO4)3
- Conclusions
- Data availability
- Author contributions
- Competing interests
- Disclaimer
- Special issue statement
- Acknowledgements
- Review statement
- References
- Supplement
- Abstract
- Introduction
- Appearance and physical properties
- Chemical analysis
- X-ray diffraction analysis
- The crystal structure
- Synthetic Na3Al(SO4)3
- Conclusions
- Data availability
- Author contributions
- Competing interests
- Disclaimer
- Special issue statement
- Acknowledgements
- Review statement
- References
- Supplement